Abstract
Thrombospondins (TSPs) are secreted multimeric glycoproteins that modulate extracellular matrix structure and cell behaviour. TSPs are involved in platelet aggregation, cell adhesion, migration, angiogenesis, wound healing, and proliferation. The purpose of this study was to investigate the function of TSP-2 on the invasion and migration of CT-26, and to determine the domain that is responsible for these functions. The antisense cDNA of TSP-2 was transfected to CT-26 (CT-26/AS-pREP4), which underexpressed TSP-2. Reduction of TSP-2 expression resulted in decreased activity, and decreased expression of the plasminogen/plasmin system. All of which resulted in decreased invasion and migration in vitro. The panels of murine TSP-2 cDNA subunits in CT-26/AS-pREP4 were established. The transfectants containing type 3 repeats domain revealed an increased in vitro invasion and migration, and increased pulmonary metastasis when inoculated into the tail vein of a syngenic mouse. The type 3 repeats domain was both integrin αvβ3 and phosphatidylinositol (PI) 3-kinase/Akt dependent. These findings may be useful for the development of therapeutic interventions that block either integrin αvβ3 or PI 3-kinase dependent Akt phosphorylation, resulting in the reduction of plasminogen/plasmin system and consequently block cell invasion, migration, and metastatic spread of colon cancer cells.
Colorectal cancer is a fatal malignancy in the United States; and its incidence is increasing in Korea.1 Advancedstage colorectal cancer may metastasize to distant sites, which most commonly involves the liver. Once the cancer spreads to distant sites, there is no effective treatment. The ability of cancer cells to metastasize to other organs and establish secondary sites of tumor formation is the main reason for treatment failure in malignancy. The processes involved in metastatic spread include; adhesion to and invasion of the basement membrane, passage through the extracellular matrix (ECM), migration through the capillaries or lymphatics, extravasation, metastatic deposits, and growth at the secondary site. Proteolysis of the ECM by the cancer cells and invasion into the ECM constituents may facilitate the escape of metastatic cells from the primary site and the subsequent invasion into the distal organs.
Thrombospondins (TSPs) are large, secreted multimeric glycoproteins that modulate ECM structure and cell behavior. There are five TSPs in vertebrates. TSP-1 and TSP-2 are homotrimers, whereas TSP-3, TSP-4, and TSP-5 are all homopentamers.2 Within the TSP family, TSP-1 and TSP-2 are the most closely related in terms of domain organization, degree of sequence homology, and function. TSP-1 and TSP-2 consist of an N-terminal domain (N), a coiled-coil oligomerisation domain (O), a procollagen module (P), three type 1 domain (T), three type 2 or EGF-like domain (E), seven calcium-binding type 3 repeats domain (X), and a C-terminal domain (C).3
TSP-2 is a calcium-sensitive, disulfide-bonded trimer.4 TSPs are involved in platelet aggregation, cell adhesion, migration, angiogenesis, wound healing, and proliferation.3 TSP-1 and TSP-2 have been identified as anti-angiogenic and anti-proliferative.5 However there are controversies on the effect of TSP-1 and TSP-2 in tumor metastasis. Treatment of TSP-1-null mice with recombinant proteins that contain the type-1 repeats of TSP-1 resulted in increased metastasis of breast cancer.6 TSP-1 was associated with increased tumor cell invasion through a transforming growth factor beta-1, urokinase-type plasminogen activator (uPA), urokinase-type plasminogen activator receptor (uPAR), and plasminogen activator inhibitor type 1 (PAI-1) in in vitro studies of breast cancer,7,8 pancreatic cancer,9 and lung cancer.10 The migration of cancer cells induced by TSP-1 was inhibited by an TSP antibody8,10 and antibody to the C-terminal domain of TSP-1.11 These findings support the pro-metastatic property of TSP-1. However, TSP-2 was under-expressed in supraglottic squamous cell carcinomas with lymph node metastases compared with those without lymph node metastases.12 In the isogenic model of spontaneous human breast cancer metastasis, high expression of TSP-1 was associated with non-metastatic phenotype.13 Transfection of TSP-1 cDNA into a human breast carcinoma cell line, MDA-MB-435 reduced pulmonary metastatic potential of the cancer.14
The objective of this study was to investigate the activity of TSP-2 in invasion and migration of CT-26, and to determine the subunit(s) of TSP-2 responsible for invasion and migration. In addition, we investigated the signal transduction pathway of TSP-2 involved in increased invasion and migration. We found that X of TSP-2 is associated with increased invasion and migration both in vitro and in vivo. In addition, the function of X was both integrin αvβ3 and phosphatidylinositol (PI) 3-kinase/Akt dependent. Furthermore, X increased the invasion and migration through over-expression of the urinary plasminogen activator (uPA), urokinase-type plasminogen activator receptor (uPAR) and plasminogen activator inhibitor-1 (PAI-1).
The cDNA subunits of murine TSP-2 (mTSP-2) were amplified from RNA of CT-26 by RT-PCR with synthetic primers (Fig. 1, Table 1). To amplify the insert of antisense (AS) vector, the forward primer contained a site for KpnI endonuclease in addition to the 23 bases of the mTSP-2 cDNA and the reverse primer contained a NotI endonuclease site and bases complementary to the mTSP-2 cDNA according to the NCBI's sequence (NM_011581). The resulting RT-PCR product was then cleaved with the endonucleases (New England BioLabs, Beverly, MA) and ligated into the corresponding sites of the pREP4 vector (Invitrogen, Carlsbad, CA) in the antisense orientation to produce AS-pREP4.
The sequences of mTSP-2 subunits were amplified with synthetic primers, and ligated into the pcDNA3.1/V5-His-TOPO vector (Invitrogen) to construct the mTSP-2 subunit(s) expression vectors. They were designated as N-pcDNA3.1, OP-pcDNA3.1, T-pcDNA3.1, E-pcDNA3.1, X-pcDNA3.1, C-pcDNA3.1, NOPTE-pcDNA3.1, and NOPTEX-pcDNA3.1. The DNA sequences of the constructs were confirmed by sequencing. The constructed vectors used for transfection were purified using affinity columns (Qiagen, Hilden, Germany) according to the manufacturer's instruction.
CT-26 (Korean Cell Line Bank, Seoul, Republic of Korea), a mouse colon cancer cell line, was maintained in DMEM with 10% fetal bovine serum (FBS), penicillin and streptomycin (Invitrogen). CT-26 cells were transfected with the pREP4 (empty vector) or AS-pREP4 using Fugene 6 (Roche, Mannheim, Germany) according to the manufacturer's protocol. Stable transfectants were selected by growing the cells in 700 µg/ml of Hygromycin B (Invitrogen), and the survived cells were maintained in 140 µg/ml of Hygromycin B for subsequent experiments. They were designated as CT-26/pREP4 and CT-26/AS-pREP4, respectively. At least 10 transfectants were selected from each vector.
The CT-26/AS-pREP4 was transfected with pcDNA3.1/V5-His-TOPO (empty vector), N-pcDNA3.1, OP-pcDNA3.1, T-pcDNA3.1, E-pcDNA3.1, X-pcDNA3.1, C-pcDNA3.1, NOPTE-pcDNA3.1, and NOPTEX-pcDNA3.1, using Fugene 6 (Roche) respectively. At least 10 stable transfectants were selected by growing the cells in 700 µg/ml of G418 (Invitrogen) in each group, and were maintained in both 140 µg/ml of G418 and 140 µg/ml of Hygromycin B for subsequent experiments. They were designated as CT-26/AS-pREP4/pcDNA3.1, CT-26/AS-pREP4/N, CT-26/AS-pREP4/OP, CT-26/AS-pREP4/T, CT-26/AS-pREP4/E, CT-26/AS-pREP4/X, CT-26/AS-pREP4/C, CT-26/AS-pREP4/NOPTE, and CT-26/AS-pREP4/NOPTEX, respectively. One or two clones from each group were used for further study.
The pDEST17-X plasmid encoding 6×His-X was constructed by Gateway Technology (Invitrogen) using pEntr3C-X, which was constructed by insertion of EcoRI and XhoI double-digested fragment of X-pcDNA3.1 into the pEntr3C vector (Invitrogen). The 6×His-X protein was expressed in E. coli BL21 (DE3) cells, and was affinity-purified using a nickel-containing affinity column, according to the manufacturer's recommendation (Novagen, Madison, WI) (Fig. 2). The eluted protein was dialyzed against phosphate-buffered saline, and the protein concentration was determined using the Bradford reagent (Bio-Rad, Hercules, CA).
The cells were grown to 80% confluence, and whole cell extracts were prepared in lysis buffer [50 mM Tris-HCl (pH 7.5), 150 mM NaCl, 1% NP-40, 1 mM EDTA, 1 mM phenylmethylsulfonyl fluoride, 1 µg/ml leupeptin, 1 µg/ml aprotinin (Sigma, St. Louis, MO), and 1× protease inhibitor cocktail (Roche)]. When needed, r-X (0.02 µg/ml), anti-integrin αvβ3 antibody (Santa Cruz Biotechnology, Santa Cruz, CA, 20 µg/ml), LY294002 (Calbiochem, San Diego, CA, 50 µM), or PD98059 (Calbiochem, 50 µM) were added during cell culture. Equal amounts of the protein were determined by a Bradford reagent and separated onto a 10% SDS-polyacrylamide gel and transferred to a polyvinylidene difluoride membrane (Millipore, Bedford, MA). The membrane was blocked with 5% skim milk for 2 hr, and then it was incubated for 16 hr with antibodies. The antibodies used included the following: anti-TSP-2 antibody (BD Biosciences, San Diego, CA.,USA), anti-uPA antibody (Santa Cruz Biotechnology), anti-uPAR antibody (Santa Cruz Biotechnology), anti-PAI-1 antibody (Santa Cruz Biotechnology), anti-Akt antibody (Cell signaling technology, Beverly, MA), anti-phospholylated Akt antibody (anti-p-Akt, Ser473, Cell signaling technology), anti-ERK1/2 antibody (Chemicon international, Temecula, CA), anti-phospho-ERK1/2 antibody (anti-p-ERK1/2, Santa Cruz Biotechnology), and anti-α-tubulin antibody (Santa Cruz Biotechnology). After washing, the membrane was incubated with the appropriate horseradish peroxidase-conjugated secondary antibody. The ECL system (Amersham Pharmacia, Buckinghamshire, U.K.) was then used to detect signals.
Cells (1×105) were grown in 60-mm plates until they were subconfluent, and then were washed three times with ice-cold phosphate buffered saline. One ml of serum-free medium was added to each plate, and the cells were incubated at 37℃. The conditioned media were collected at 72 hr, and clarified by centrifugation. The proteins from each conditioned medium were assessed by the Bradford assay.
For the determination of the plasminogen activators, whole cell extracts were analyzed on 10% SDS polyacrylamide gels containing 1 mg/ml α-casein (Sigma) and 10 µg/ml plasminogen (Calbiochem). After electrophoresis, gels were incubated overnight at room temperature in 100 mM glycine buffer with 5 mM EDTA at pH 8.0. Caseinolytic activity resulting from plasminogen activation was noted by white lysis zones after gel staining with G250 Coomassie brilliant blue.15
In vitro invasion assay was performed using a Transwell chamber and 8 µm pore size polycarbonate membranes (Costar, Cambridge, MA) coated with reconstituted ECM (Matrigel; Becton Dickson, Bedford, MA). Briefly, the cells were seeded at a density of 5×104 cells in 200 µl of serum-free DMEM in the upper compartment of the Transwell, and allowed to invade the polycarbonate membrane for 24 hr. The lower chamber was filled with DMEM that contained 10% FBS. The non-invaded cell portions on the upper surface of the membrane were removed from the chamber, and the portions with invaded cells on the lower surface of the membrane were stained with Diff-Quick (Kokusai Shiyaku, Kobe, Japan). The numbers of invaded cells were counted in four randomly selected microscopic fields (×100) per membrane. The r-X (0.02 µg/ml), anti-integrin αvβ3 antibody (20 µg/ml), LY294002 (50 µM), and PD98059 (50 µM) were added in the upper compartment. The procedure for the in vitro migration assays was identical to that used for the in vitro invasion assays with the exception that the 8 µm pore size polycarbonate membranes were not coated with ECM.
The Animal Research and Ethics Board of Chonnam National University Medical School reviewed and approved all protocols for the animal studies. The care and use of the animals were in accordance with the institution's guidelines. Eight-week-old male BALB/c mice (Daehan Biolink, Emsung, Republic of Korea) were used. Animals were given food and water ad libitum. They were kept in a room with constant temperature and humidity. All mice were adapted to our laboratory conditions for one week before starting the experiments. They were randomized into five groups based on mean body weight and were initially housed in up to 10 mice per group.
Subconfluent cells were harvested by trypsinization, and were resuspended in DMEM without serum at a density of 4×106 cells/ml. The cells (1.6×105 cells in 100 µl of PBS) were injected into the lateral tail vein of the mice with a 26-gauge needle.16 On 14th day after tail vein injection, the animals were sacrificed by cervical dislocation. The lungs were excised en bloc, and were immersed in 10% buffered formalin. The lung weight was measured, and the number of surface pulmonary nodules was counted under the dissecting microscope. The lung tissue was processed for histological examination.
The empty vector transfected CT-26 (CT-26/pREP4) showed slightly altered TSP-2 expression when compared with the CT-26. However AS vector transfected CT-26 (CT-26/AS-pREP4) revealed 20% and 40% of TSP2 expression compared with CT-26/pREP4 in the whole cell extracts (Fig. 3A) and serumless conditioned media (Fig. 3B), respectively. The CT-26/AS-pREP4 revealed lower gelatinolytic activity (Fig. 3C), and decreased expression of uPA, uPAR, and PAI-1 than CT-26/pREP4 (Fig. 3D). Down-regulation of TSP-2 in CT-26/AS-pREP4 was associated with the decreased activity of uPA, and the decreased expression of uPA, uPAR, and PAI-1.
The uPA expression was slightly changed in CT-26/AS-pREP4/NOPTEX-1, CT-26/AS-pREP4/NOPTEX-2, CT-26/AS-pREP4/X-1, and CT-26/AS-pREP4/X-2 when compared with CT-26/pREP4. However it was significantly decreased in CT-26/AS-pREP4, CT-26/AS-pREP4/pcDNA3.1, CT-26/AS-pREP4/NOPTE-1, and CT-26/AS-pREP4/ NO-PTE-2 (Fig. 4A). The uPA activity measured by casein/plasminogen zymography revealed a similar pattern as western blot (Fig. 4B). The findings suggested that the X might be associated with the uPA expression and activity. The in vitro invasion of CT-26/AS-pREP4 and CT-26/AS-pREP4/NOPTE-1 was significantly lower than that of CT-26/pREP4. In addition, invasion of CT-26/AS-pREP4/NO-PTEX-2 and CT-26/AS-pREP4/X-2 was significantly higher than that of CT-26/AS-pREP4 and CT-26/AS-pREP4/NOPTE-1, and was as high as that of CT-26/pREP4. The in vitro migration assay disclosed the similar trend as in vitro invasion assay (Fig. 4C, D). The findings indicated that the X was associated with increased in vitro invasion and migration of CT-26/ AS-pREP4.
Western blot analyses, in vitro invasion assay, and in vitro migration assay were performed in CT-26/AS-pREP4 in order to find the signal transduction pathway involved in the X induced upregulation of uPA, uPAR, and PAI-1. When r-X (0.02 µg/ml) was treated in CT-26/AS-pREP4. the phosphorylated Akt (p-Akt) level increased threefold at 5 min until 240 min, while the unphosphorylated Akt remained unchanged. Both phosphorylated and unphosphorylated Akt remained unaltered until 240 min when either anti-integrin αvβ3 antibody (20 µg/ml) or LY294002 (50 µM) was added prior to r-X (0.02 µg/ml) treatment (Fig. 5A). When r-X (0.02 µg/ml) was added in CT-26/AS-pREP4, both phosphorylated ERK1/2 (p-ERK1/2) and unphosphorylated ERK1/2 remained constant until 240 min. This result indicated that the X was not MAP kinase/ERK kinase (MEK) dependent (Fig. 5B). The uPA level of in CT-26/AS-pREP4 increased twofold after 60 min of r-X (0.02 µg/ml) treatment until 240 min. When we added either anti-integrin αvβ3 antibody (20 µg/ml) or LY294002 (50 µM) prior to r-X (0.02 µg/ml) treatment, the uPA level remained unaltered until 240 min. However, pretreatment of PD98059 (50 µM) prior to r-X (0.02 µg/ml) treatment could not block the effect of r-X on the uPA level (Fig. 5C). The effect of r-X (0.02 µg/ml) on the uPAR and PAI-1 expression was similar as that on uPA (Fig. 5D, E). The results indicated that the X induced up-regulation of the plasminogen/plasmin system through integrin αvβ3 and PI 3-kinase/Akt pathway.
In vitro invasion of CT-26/AS-pREP4 was increased more than twofold when r-X (0.02 µg/ml) was added. However addition of either anti-integrin αvβ3 antibody (20 µg/ml) or LY294002 (50 µM) prior to r-X treatment significantly decreased invasion of CT-26/AS-pREP4 compared to r-X (0.02 µg/ml) only treatment. However PD98059 (50 µM) application prior to r-X (0.02 µg/ml) treatment did not block the effect of r-X (Fig. 6A). The in vitro migration assay revealed a similar tendency as the in vitro invasion assay (Fig. 6B). This finding displayed a key role for the PI 3-kinase/Akt pathway in the X induced in vitro invasion and migration.
In order to verify that transfection of the X increased metastasis of CT-26/AS-pREP4, we inoculated the transfectants into the tail vein of BALB/c mice. They were CT-26/pREP4, CT-26/AS-pREP4, CT-26/AS-pREP4/NOPTE-1, CT-26/AS-pREP4/NOPTEX-2, and CT-26/AS-pREP4/X-2, respectively. Four mice died just after inoculation; one in CT-26/pREP4 inoculated group, one in CT-26/AS-pREP4 inoculated group, and two in CT-26/AS-pREP4/X-2 inoculated group, respectively. The lungs at 14 days after inoculation were solid and were covered with many surface nodules in CT-26/pREP4, CT-26/AS-pREP4/NOPTEX-2, and CT-26/AS-pREP4/X-2 inoculated group. In CT-26/AS-pREP4 and CT-26/AS-pREP4/NOPTE-1 inoculated group, the lungs were soft and only a few surface nodules were observed (Fig. 7A). The microscopic findings were consistent with the gross morphologies (Fig. 7B). The number of lung surface nodules of CT-26/pREP4 inoculated group was 339±216 (mean±standard deviation, n=9), CT-26/AS-pREP4 48±70 (n=9), CT-26/AS-pREP4/NOPTE-1 11±13 (n=10), CT-26/AS-pREP4/NOPTEX-2 254±223 (n=10), and CT-26/AS-pREP4/X-2 484±181 (n=8), respectively. Both CT-26/AS-pREP4 and CT-26/AS-pREP4/NOPTE-1 inoculated groups revealed a significantly decreased number of lung surface nodules compared to CT-26/pREP4 inoculated, CT-26/AS-pREP4/NOPTEX-2 inoculated, and CT-26/AS-pREP4/X-2 inoculated groups. CT-26/AS-pREP4/X-2 inoculated group showed more lung surface nodules than CT-26/AS-pREP4/NOPTEX-2 inoculated group (Fig. 7C). The lung weight of CT-26/pREP4 inoculated group was 0.46±0.16 g (mean±standard deviation, n=9), CT-26/AS-pREP4 0.25±0.05 g (n=9), CT-26/AS-pREP4/NOPTE-1 0.26±0.05 g (n=10), CT-26/AS-pREP4/NOPTEX-2 0.32±0.06 g (n=10), and CT-26/AS-pREP4/X-2 0.63±0.21 g (n=8), respectively. The lung weight revealed the same inclination as the number of lung surface nodules (Fig. 7D). Taken together, the results indicated that transfectants containing X revealed increased pulmonary metastases in BALB/c mice.
This study reveals that reduction of TSP-2 in CT-26 cells is associated with decreased invasion, migration and metastasis. At 14 days after intravenous inoculation into the tail vein of syngenic mice, CT-26/AS-pREP4 with low TSP-2 expression formed fewer nodules on the lung surface, which was in contrast to the vector-transfected CT-26/pREP4 that produced many nodules. This finding is consistent with the pro-metastatic effect of TSP-1 or -2 in breast cancer,7,8 lung cancer,10 and pancreatic cancer.9 Our results, using transfection of TSP-2 subunits in CT-26/AS-pREP4, demonstrated that X was associated with increased uPA activity and uPA expression. The seven X account for the most highly conserved region, both among the different types of TSP from different species and among different family members from the same species. The functions of the X include cell spreading and cytoskeletal organization,17 chemotaxis,18 cell attachment,19 platelet aggregation,20 and phagocytosis.21
Our data show that the effect of the X on invasion and migration is integrin αvβ3 dependent. Moreover, pretreatment of these cells with anti-αvβ3 integrin antibody followed by treatment with r-X suppressed the Akt phosphorylation. This suggests that upregulation of X induces the PI 3-kinase/Akt activity through the αvβ3 integrin-mediated pathway. The X of TSP-1 and TSP-2 orthologues, contains a conserved RGD sequence, the integrin-binding motif.22 Binding of the RGD sequence with integrin αvβ3, mediates the adhesion of platelets23 and chemotaxis in human vascular smooth muscle cells.18 It mediates tyrosine phosphorylation of both focal adhesion kinase, and the p85 regulatory subunit of PI 3-kinase.24 Treatment with LY294002 blocked the effect of the X on invasion and migration of CT-26/AS-pREP4. This finding confirmed a key role for the PI 3-kinase/Akt-mediated pathway in invasion and migration induced by the X. Cell migration is often associated with the activation of PI 3-kinase.25 One of the downstream target molecules of PI 3-kinase is Akt. A serine threonine kinase is activated by factors that stimulate PI 3-kinase activity in cells such as thrombin, platelet-derived growth factor, and insulin. In the PI 3-kinase dependent pathway, PI 3,4-biphosphate, a product of PI 3-kinase directly binds to the pleckstrin homology domain of Akt and leads to its activation. Several metastatic tumors express higher levels of Akt.26
MAP kinase has also been implicated in TSP-1 mediated migration. The Ras-Raf-MEK1/2 cascade can activate ERK1/2 when integrin binds to ECM. However, our data showed that the ERK1/2 pathway was not involved in the X induced invasion and migration of CT-26/AS-pREP4. This is consistent with the results from human vascular smooth muscle cells, which demonstrated that RGD-containing peptide does not affect tyrosine phosphorylation of ERK1/2.18
The plasminogen/plasmin system is one of the main protease systems involved in cancer progression. After binding to uPAR, uPA is activated and subsequently converts plasminogen to plasmin. uPA catalyzes the conversion of the inactive zymogen plasminogen to the active broad-spectrum plasmin. This will then degrade a number of matrix proteins and activate other proteases, including some matrix metalloproteinases.27 It has been shown that adhesion and invasion of carcinoma cells are potentiated by TSP-1 or -2 through a mechanism involving the plasminogen activator system. This activity is due to the TSP-1 or -2-mediated up-regulation of uPA, uPAR and PAI-1.7,8,10 In addition to increased invasion and migration, TSP-1 and -2 inhibit angiogenesis and tumor growth in a variety of tumors.28 The anti-angiogenetic activity of TSP-1 has been mapped to TSP type 1 repeats,29 and interaction of TSP type 1 repeats with CD36 has been implicated also.30 In addition to CD 36 and integrins, TSP-1 and -2 interact with integrin-associated protein, low density lipoprotein receptor-related protein, proteoglycans, TGF-β1, platelet derived growth factor, and fibroblast growth factor 2. The activity of TSP-1 and -2 may be determined through their interaction with binding partners. Further study is needed to determine the mechanism involved in the interaction of the TSP with their binding partners.
In conclusion, we have demonstrated that the X of TSP-2 increased invasion, migration, and metastasis of CT-26 cells. The function of the X in increased invasion and migration was integrin αvβ3 dependent. The repeats increased expression of uPA, uPAR, and PAI-1 through tyrosine phosphorylation of PI 3-kinase/Akt. These findings may be useful for the development of novel therapeutic interventions that can block the X-regulated PI 3-kinase dependent Akt phosphorylation resulting in reduction of uPA, uPAR, and PAI-1 expression and consequently block cell invasion, migration and metastatic spread of colon cancer cells.
We have demonstrated that the X of TSP-2 was involved in invasion, migration, and metastasis of CT-26 cells. We also found the function was both integrin αvβ3 and PI 3-kinase/Akt dependent which consequently upregulated uPA, uPAR, and PAI-1. The findings may be useful for the development of novel therapeutic interventions that block invasion, migration and metastatic spread of colon cancer cells.
Figures and Tables
Fig. 1
Schematic representation of the domains of mTSP-2 and its subunits constructed in vectors. N, NH2-terminal domain; O, oligomerisation sequence; P, procollagen module; T, type 1 repeats; E, EGF like; X, type 3 repeats domain; C, C-terminal globule; AS, antisense cDNA. Arrow indicates the orientation of insert.
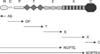
Fig. 2
The His-THBS2 fusion protein was eluted and immunoblotted using the anti-His antibody (arrow).
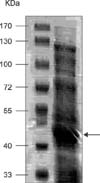
Fig. 3
Down-regulation of TSP-2 results in decreased uPA activity, and decreased uPA, uPAR and PAI-1 expression. (A) Western blot of whole cell extracts reveals less expression of TSP-2 in CT-26/AS-pREP4 than in CT-26 or CT-26/pREP4. Western blot of α-tubulin reveals equal loading of protein. (B) Western blot of excreted TSP-2 in serumless conditioned medium reveals endogenous TSP-2 expression in CT-26 and CT-26/pREP4, while decreased expression in CT-26/AS-pREP4. (C) Casein/plasminogen zymography shows decreased uPA activity in CT-26/AS-pREP4 than in CT-26 or CT-26/pREP4. (D) Western blot of CT-26/AS-pREP4 reveals reduced expression of uPA, uPAR, and PAI-1 compared to CT-26 or CT-26/pREP4.
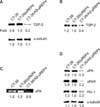
Fig. 4
The X of TSP-2 is associated with increased uPA expression and uPA activity. (A), (B) Western blot of uPA (A) and casein/plasminogen zymography (B) of the transfectants of mTSP-2 subunits transfected in CT-26/AS-pREP4. Two clones (e.g.; CT26/AS-pREP4/X-1 and CT26/AS-pREP4/X-2) per each transfectants were examined in this assay. Transfectants that contain the X retained uPA expression and uPA activity compared to parental cells, while those that do not have the X reveal very weak uPA expression and uPA activity. Lane 1, CT-26; 2, CT-26/pREP4; 3, CT-26/AS-pREP4; 4, CT-26/AS-pREP4/pcDNA3.1; 5, CT-26/AS-pREP4/NOPTEX; 6, CT-26/AS-pREP4/NOPTEX; 7, CT-26/AS-pREP4/NOPTE; 8, CT-26/AS-pREP4/NOPTE; 9, CT-26/AS-pREP4/X; 10, CT-2/AS-pREP4/X. (C), (D) The in vitro invasion (C) and migration (D) of CT-26/AS-pREP4 and CT-26/AS-pREP4/NOPTE-1 are decreased compared to CT-26/pREP4; while those of CT-26/AS-pREP4/NOPTEX-2 and CT-26/AS-pREP4/X-2 are increased compared to CT-26/AS-pREP4 and CT-26/AS-pREP4/NOPTE-1. Group I, CT-26/pREP4; Group II, CT-26/AS-pREP4; Group III, CT-26/AS-pREP4/NOPTE-1; Group IV, CT-26/AS-pREP4/NOPTEX-2; Group V, CT-26/AS-pREP4/X-2. *p<0.01.
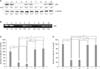
Fig. 5
Signal transduction of the r-X is both integrin αvβ3 and PI 3-kinase/Akt dependent. (A) Phopshorylated-Akt (p-AKT) is increased up to three fold after 5 min by treatment of r-X (0.02µg/ml) in CT-26/AS-pREP4, while unphosphorylated Akt remains unchanged until 240 min. Pretreatment of either anti-integrin αvβ3 antibody (20µg/ml) or LY294002 (50µM) blocks over-expression of p-Akt. Western blot of α-tubulin reveals equal loading of the proteins. (B) Treatment of r-X (0.02µg/ml) does not alter the expression of both phosphorylated (p-ERK1/2) and unphosphorylated ERK1/2 until 240 min. Western blot of α-tubulin reveals equal loading of the proteins. (C) Treatment of r-X (0.02µg/ml) increases uPA expression up to twofold after 60 min until 240 min. Pretreatment of anti-integrin αvβ3 antibody (0.02µg/ml) or LY294002 (50µM) block the effect of r-X. Pretreatment of PD98059 (50µM) does not block the effect of r-X. (D), (E) Treatment of r-X (0.02µg/ml) increases both uPAR (D) and PAI-1 (E) expression up to twofold after 60 min until 240 min. Treatment of anti-integrin αvβ3 antibody (20µg/ml) or LY294002 (50µM) block the effect of r-X.
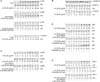
Fig. 6
r-X increases in vitro invasion and migration, which is integrin αvβ3 and PI 3-kinase/Akt dependent. (A), (B). Effect of r-X, anti-integrin αvβ3 antibody, LY294002, and PD98059 of in vitro invasion (A) and in vitro migration (B) of CT-26/AS-pREP4. Treatment of r-X (0.02 µg/ml) increases invasion and migration of CT-26/AS-pREP4. However both anti-integrin αvβ3 antibody (20 µg/ml) and LY294002 (50 µM) block the pro-invasion and pro-migration effect r-X. PD98059 (50 µM) does not block the r-X effect. The error bar represents the standard deviation. !p<0.05; †p<0.01.
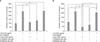
Fig. 7
Transfectants containing the X are associated with increased pulmonary metastasis. (A) Gross photographs of the lungs at 14 days after inoculation. The Roman numerals represent the group of mice, and the Arabic numerals represent each mouse in the group. The lungs are enlarged and many surface nodules are found in groups I, IV and V. Group I, CT-26/pREP4 inoculated mice; Group II, CT-26/AS-pREP4 inoculated mice; Group III, CT-26/AS-pREP4/NOPTE-1 inoculated mice; Group IV, CT-26/AS-pREP4/NOPTEX-2 inoculated mice; V, Group CT-26/AS-pREP4/X-2 inoculated mice. (B) Histological findings of the lungs demonstrates many tumor nodules are in groups I, IV and V and a few nodules in groups II and III. a, Group I; b, Group II; c, Group III; d, Group IV; e, Group V. An asterisk represents the tumor nodule. Bar represents 1 mm. Hematoxylin-eosin stain. (C), (D) The number of lung surface nodules (C) was larger in groups II and III than in groups I, IV, and V. The group V revealed more surface nodules than group IV. The lung weight (D) revealed a similar trend as the number of lung surface nodules. The error bar represents standard deviation. !p<0.05; †p<0.01.
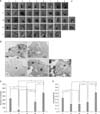
Table 1
Primer sequence for amplification of mTSP-2 cDNA subunits
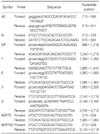
The upper case letters represent the mTSP-2 cDNA sequence or its complementary sequence according to NM_011581. For amplifying AS, the forward primer contained a site for KpnI endonuclease (lower case letters) in addition to the 23 bases of the mTSP-2 cDNA and the reverse primer contained a NotI endonuclease site (lower case letters)and bases complementary to the mTSP-2 cDNA. Nomenclature: AS, antisense; N, N-terminal; O, oligomerisation sequence; P, procollagen module; T, type 1repeats; E, EGF-like; X, type 3 repeats; C, C-terminal globule.
Acknowledgements
This study was supported by a grant from the National R&D Program for Cancer Control, Ministry of Health & Welfare, Republic of Korea (HCRE 08028-6). YJK received the grant of Chonnam National University Hospital Research Institute of Clinical Medicine (CNUHRICM-U-200309).
References
1. Bae JM, Jung KW, Won YJ. Estimation of cancer deaths in Korea for the upcoming years. J Korean Med Sci. 2002. 17:611–615.


3. O'Rourke KM, Laherty CD, Dixit VM. Thrombospondin 1 and thrombospondin 2 are expressed as both homo- and heterotrimers. J Biol Chem. 1992. 267:24921–24924.
4. Sargiannidou I, Qiu C, Tuszynski GP. Mechanisms of thrombospondin-1-mediated metastasis and angiogenesis. Semin Thromb Hemost. 2004. 30:127–136.


5. Lawler J. Thrombospondin-1 as an endogenous inhibitor of angiogenesis and tumor growth. J Cell Mol Med. 2002. 6:1–12.


6. Yee KO, Connolly CM, Duquette M, Kazerounian S, Washington R, Lawler J. The effect of thrombospondin-1 on breast cancer metastasis. Breast Cancer Res Treat. 2009. 114:85–96.


7. Albo D, Berger DH, Wang TN, Hu X, Rothman V, Tuszynski GP. Thrombospondin-1 and transforming growth factor-beta l promote breast tumor cell invasion through up-regulation of the plasminogen/plasmin system. Surgery. 1997. 122:493–500.


8. Arnoletti JP, Albo D, Granick MS, Solomon MP, Castiglioni A, Rothman VL, et al. Thrombospondin and transforming growth factor-beta 1 increase expression of urokinase-type plasminogen activator and plasminogen activator inhibitor-1 in human MDA-MB-231 breast cancer cells. Cancer. 1995. 76:998–1005.


9. Albo D, Berger DH, Vogel J, Tuszynski GP. Thrombospondin-1 and transforming growth factor beta-1 upregulate plasminogen activator inhibitor type 1 in pancreatic cancer. J Gastrointest Surg. 1999. 3:411–417.


10. Albo D, Arnoletti JP, Castiglioni A, Granick MS, Solomon MP, Rothman VL, et al. Thrombospondin (TSP) and transforming growth factor beta 1 (TGF-beta) promote human A549 lung carcinoma cell plasminogen activator inhibitor type 1 (PAI-1) production and stimulate tumor cell attachment in vitro. Biochem Biophys Res Commun. 1994. 203:857–865.


11. Yabkowitz R, Mansfield PJ, Dixit VM, Suchard SJ. Motility of human carcinoma cells in response to thrombospondin: relationship to metastatic potential and thrombospondin structural domains. Cancer Res. 1993. 53:378–387.
12. Bai W, Wang L, Ji W, Gao H. Expression profiling of supraglottic carcinoma: PTEN and thrombospondin 2 are associated with inhibition of lymphatic metastasis. Acta Otolaryngol. 2008. 129:569–574.


13. Urquidi V, Sloan D, Kawai K, Agarwal D, Woodman AC, Tarin D, et al. Contrasting expression of thrombospondin-1 and osteopontin correlates with absence or presence of metastatic phenotype in an isogenic model of spontaneous human breast cancer metastasis. Clin Cancer Res. 2002. 8:61–74.
14. Weinstat-Saslow DL, Zabrenetzky VS, VanHoutte K, Frazier WA, Roberts DD, Steeg PS. Transfection of thrombospondin 1 complementary DNA into a human breast carcinoma cell line reduces primary tumor growth, metastatic potential, and angiogenesis. Cancer Res. 1994. 54:6504–6511.
15. Huet E, Brassart B, Cauchard JH, Debelle L, Birembaut P, Wallach J, et al. Cumulative influence of elastin peptides and plasminogen on matrix metalloproteinase activation and type I collagen invasion by HT-1080 fibrosarcoma cells. Clin Exp Metastasis. 2002. 19:107–117.
16. Kobayashi H, Gonda T, Uetake H, Higuchi T, Enomoto M, Sugihara K. JTE-522, a selective COX-2 inhibitor, interferes with the growth of lung metastases from colorectal cancer in rats due to inhibition of neovascularization: a vascular cast model study. Int J Cancer. 2004. 112:920–926.


17. Anilkumar N, Annis DS, Mosher DF, Adams JC. Trimeric assembly of the C-terminal region of thrombospondin-1 or thrombospondin-2 is necessary for cell spreading and fascin spike organisation. J Cell Sci. 2002. 115:2357–2366.


18. Lymn JS, Patel MK, Clunn GF, Rao SJ, Gallagher KL, Hughes AD. Thrombospondin-1 differentially induces chemotaxis and DNA synthesis of human venous smooth muscle cells at the receptor-binding level. J Cell Sci. 2002. 115:4353–4360.


19. Adams J, Lawler J. Extracellular matrix: the thrombospondin family. Curr Biol. 1993. 3:188–190.
20. Bonnefoy A, Hantgan R, Legrand C, Frojmovic MM. A model of platelet aggregation involving multiple interactions of thrombospondin-1, fibrinogen, and GPIIbIIIa receptor. J Biol Chem. 2001. 276:5605–5612.


21. Savill J, Hogg N, Ren Y, Haslett C. Thrombospondin cooperates with CD36 and the vitronectin receptor in macrophage recognition of neutrophils undergoing apoptosis. J Clin Invest. 1992. 90:1513–1522.


22. Ruoslahti E. RGD and other recognition sequences for integrins. Annu Rev Cell Dev Biol. 1996. 12:697–715.


23. Lawler J, Weinstein R, Hynes RO. Cell attachment to thrombospondin: the role of ARG-GLY-ASP, calcium, and integrin receptors. J Cell Biol. 1988. 107:2351–2361.


24. Lymn JS, Rao SJ, Clunn GF, Gallagher KL, O'Neil C, Thompson NT, et al. Phosphatidylinositol 3-kinase and focal adhesion kinase are early signals in the growth factor-like responses to thrombospondin-1 seen in human vascular smooth muscle. Arterioscler Thromb Vasc Biol. 1999. 19:2133–2140.


25. Sliva D, Rizzo MT, English D. Phosphatidylinositol 3-kinase and NF-kappaB regulate motility of invasive MDA-MB-231 human breast cancer cells by the secretion of urokinase-type plasminogen activator. J Biol Chem. 2002. 277:3150–3157.


26. Bellacosa A, Testa JR, Staal SP, Tsichlis PN. A retroviral oncogene, akt, encoding a serine-threonine kinase containing an SH2-like region. Science. 1991. 254:274–277.


27. Morgan H, Hill PA. Human breast cancer cell-mediated bone collagen degradation requires plasminogen activation and matrix metalloproteinase activity. Cancer Cell Int. 2005. 5:1.
28. Streit M, Riccardi L, Velasco P, Brown LF, Hawighorst T, Bornstein P, et al. Thrombospondin-2: a potent endogenous inhibitor of tumor growth and angiogenesis. Proc Natl Acad Sci U S A. 1999. 96:14888–14893.

