Abstract
The aim of this study was to ascertain the folate receptor (FR) targetability by an in vitro study and to acquire FR-targeted images in vivo models by using synthetic folate conjugates. PEG-folate was synthesized and labeled with 99mTc and fluorescein isothiocynate (FITC). Cell uptake studies were carried out in KB cells (FR-positive) and A549 cells (FR-negative) using FITC- and the 99mTc-labeled conjugates. The radiolabeled conjugate was intravenously injected to KB tumor xenografted mice. After it was injected, gamma images were recorded at 30 min, 1, 2, 3 and 4 hr. Cell uptake studies showed a difference between the KB cells and the A549 cells by flow cytometry analysis and gamma counting. On in vivo images, the tumor-to-normal muscle ratio was greater than 4. It ascertained that the PEG-folate conjugate specifically binds to the FR expressed on tumor cells in vitro. Moreover, it was possible to acquire the FR-targeted gamma images using PEG-folate conjugates in tumor models.
Folic acid has a high affinity with folate receptor (FR) and is known to be a cellular membrane glycoprotein with a molecular weight of approximately 38-40 kDa (1); it shows a different binding affinity for folic acid in many types of tumors. Drug delivery systems containing anti-cancer agents have recently received much attention due to their unique accumulation behavior at folate-positive tumor sites (2, 3). Folic acid binds to the folate receptors at cell surfaces with a very high affinity (Kd=-1 nM), and it is internalized by receptor-mediated endocytosis (4). Additionally, folate receptor has been associated with the parameters of biological aggressiveness in different cancers. Folate receptor was over-expressed to a large degree in ovarian neoplasmas that had a high histological grade, advanced stage, and serious histology. A multiple comparison analysis showed that the folate receptor expression of stage IV and omental metastases was significantly higher than that in early stage disease (5). High frequencies of receptor overexpression have been found in many types of tumors, including ovarian, endometrial, colorectal, breast, lung, renal cell carcinomas, brain metastases derived from epithelial cancers, and neuroendocrine carcinomas (6). So this receptor has been recognized as a tumor marker, and folate has been conjugated with anti-cancer drugs or liposomes for tumor targeting.
The main condition of radio-labeled conjugates is needed some characteristics such as binding specificity to ligand, nontoxicity, and high water solubility with adequate circulating time into blood. Bi-functional Poly (ethylene glycol) is generally a very hydrophilic molecule, and it helps achieve a long circulation time. A wide range of targeting moieties such as galactose, folate, cell adhesive peptides, epidermal growth factor, and transferrin have been directly conjugated onto the distal end of the PEG chain (7). Folate modified PEG selectively binds with tumor cells and this leads to receptor-mediated endocytosis (8). The other amine group and the flexibility of Jeffamine have the possibility of being labeled with 99mTc. Thus, we tried to molecularly target tumors and obtain their in vivo images by using bi-functional PEG.
In this study, PEG-folate, which can specifically bind to tumor, was conjugated and was then labeled with 99mTc. We analyzed the flow cytometric data and fluorescence images that depended on the degree of the folate receptor expression by using PEG-folate-FITC in vitro. We performed experiments that showed cellular uptake of the radio-labeled conjugate. For imaging an animal model, we first tested the labeling efficiency of 99mTc PEG-folate and its stability in human serum. Gamma camera images were acquired by using FR-positive mice models.
The carboxylate group of folic acid was activated by N-hydroxysuccimide (NHS) and dicyclohexylcarbodiimide (DCC) (9). Briefly, folic acid (0.11 g, 250 µM) and triethylamine dissolved in dimethylsulfoxide (DMSO, 2 mL) were reacted with NHS (0.057 g, 500 µM) and DCC (0.1 g, 500 µM) at room temperature for 16 hr (folic acid: NHS: DCC molar ratio= 1:2:2).
The precipitate was removed by filtration (0.22 µm). The activated folic acid was reacted with 0.5 g of Jeffamine (NH2-PEG-NH2, MW: 4000) that was dissolved in 5 mL of DMSO. The reaction was performed under a nitrogen atmosphere at room temperature for 4 days. The supernatant was diluted with deionized water, dialyzed (MWCO 3500, Spectrum) against deionized water (7-8 times) and then it was freezedried. The structure of the PEG-folate conjugate in DMSO-d6 was determined by 1H-NMR. 1H-NMR was performed on a 400 MHz apparatus (Jeol, Tokyo, Japan) and the results were analyzed by MestRe-C 2.3a. PEG-folate was dissolved in borate buffer (0.05 M, pH 8.3). Fluorescein isothiocyanate (0.005 g dissolved in DMSO anhydrous) was slowly added to the buffer and the mixture was gently stirred at room temperature for 4 hr. The FITC-PEG-folate was dialyzed and then freeze-dried.
In order to investigate selective cellular uptake of PEG-folate via folate-receptor-mediated endocytosis, KB cells and A549 cells (1×105) were incubated in culture medium that lacked folic acid for 24 hr. The cells were next treated with PEG-FITC, PEG-folate-FITC or PEG-folate-FITC with folic acid for 1 hr, and the cells were washed three times with PBS and harvested using 500 µL of PBS with BSA. Finally we analyzed the cells by flow cytometry (FACcan, Beckton-Dickinson, CA, U.S.A.) of the cells.
Collagen coated cover glasses (Iwaki, Tokyo, Japan) were placed in the wells of 24-well plates. KB cells and A549 cells were then seeded at a density of 1×104 cells/well and they were allowed to incubate in culture medium that had no folic acid for 24 hr. After 24 hr, the cells were treated with 50 µM PEG-folate-FITC for 1 hr. For the free folate competition studies, 1 mM folic acid was added to the incubation medium. After washing three times with PBS, the cell-associated fluorescent images were viewed by fluorescence microscopy (Axiovison plus II, Zwiss, Jena, Germany).
PEG-folate was dissolved in distilled water (5 µg/µL). Stannous chloride was dissolved in 0.02 N HCl (0.2 µg/µL, w/v). Two hundred micro liters of PEG-folate was mixed with 10 µL of stannous chloride solution in a vial at room temperature. Labeling was carried out by adding 37 MBq (1 mCi) sodium 99mTc pertechnetate to the above mixture, and this was gently shaken. The 99mTc pertechnetate was eluted from a technetium generator in our hospital (Daiichi Radioisotope Laboratories, Ltd. Tokyo, Japan). The mixture was allowed to react for a further 30 min, with shaking from time to time.
The stability of 99mTc PEG-folate was determined by ITLC and with using ITLC-sg strips (Gelman Sciences, NY, U.S.A.) as the stationary phase, and saline was used as the mobile phase and the stability of 99mTc PEG-folate was determined at 10 and 30 m and at 1, 2, 3, 6 and 16 hr by using a TLC scanner (Bioscan, Washington, D.C., U.S.A.). The stability in the serum was tested in 0.5 mL of human serum that was added to 200 µL of radiolabeled conjugate, and the mixture was reacted in a 37℃ water bath. The stability was determined at 10 m and 30 m, and at 1, 2, 3, 6 and 16 hr.
Labeling efficiency (%)=(Total count-Count of free pertechnetate)/Total count×100
KB cells and A549 cells (1×106) were incubated in the absence of folic acid in culture medium for 24 hr. After 24 hr of incubation, the cells were treated with 99mTc PEG-folate, 99mTc PEG or 99mTc PEG-folate with folic acid for 30 min, and then the cells were washed three times with 1× HBSS and harvested. Finally, we measured the CPM by gamma counter (Cobra II PACKARD, MN, U.S.A.) of the cells and counted the number of cells. The method and concentration were same as in the in vivo imaging study, and the radio-activity of mixture was 7.4 MBq (200 µCi/500 µL).
All the animal experiments were approved by the Chonbuk National University School of Medicine Committee, and they were performed in accordance with their guidelines. Female athymic nude mice weighing 16-18 g each (4-5 wks, Japan SLC, Inc., Tokyo, Japan) were placed on a folate-free murine diet (Dyets, PA, U.S.A.) from arrival (day 0) to day 15. The animals were inoculated subcutaneously with suspended KB cells (1×107/0.1 mL) in the left thigh region on day 16 (10-12). The radiotracer studies were carried out 3 weeks after KB cell implantation and at a total of 5 weeks after initiation of the folate-free diet.
After sedating the mice with ketamine mixed with xylazine, 99mTc PEG-folate was administered intravenously via a tail vein to the animals. We then performed scintigraphy and recorded the 30 min, 1, 2, 3 and 4 hr images by using ECAM camera (SIEMENS, NY, U.S.A.). The camera was equipped with a 3 mm pinhole collimator, the window setting was 140 keV and the width was 20%. The static images were stored in a 512×512 matrix size and the acquisition times were 180 sec. 99mTc PEG-folate and free folic acid (10 µM) were co-injected intravenously via a tail vein (n=3) to block the receptor-mediated uptake of the radiotracer. After administration, we acquired the gamma camera images in the same way as above. The scintigraphic results were analyzed by drawing the regions of interest (ROI) on the lesions and comparing them to the normal thigh muscle.
Folic acid has two α- and γ-carboxylic acids, but γ-carboxylic acid is more selectively activated due to its higher reactivity. The terminal carboxylic acid of folic acid was subsequently activated by DCC/NHS, and it reacted with the primary amine group of PEG.
The conjugate was characterized by 1H NMR spectroscopy as shown in Fig. 1. It is apparent that the spectrum of the conjugate contains signals originating from both folic acid and the PEG. The signal at 3.7 ppm corresponds to the proton of the PEG (-CH2-), the signals at 6.7 and 7.6 ppm correspond to the para-aminobenzoic acid (PABA) from the folate, and the signal at 8.7 ppm corresponds to the pteridine moiety proton from the folate. The yield of PEG-folate was 75%.
In order to investigate the selective targeting ability of PEG-folate for the FRs on the cell, KB cells and A549 cells were employed as FR-positive cancer cells and FR-negative cancer cells, respectively. As shown in Fig. 2, the results of flow cytometry of the KB cells show a greater cellular uptake of PEG-folate-FITC than that of PEG-FITC or the PEG-folate-FITC added folic acid when folic acid was absent in the culture medium (Fig. 2A). However, there was no difference in the A549 cells between PEG-folate-FITC and the PEG-FITC (Fig. 2B).
The extent of the cellular uptake of PEG-folate in KB cells (Fig. 3A, D) was significantly higher than in the A549 cells (Fig. 3C, F) when folic acid was absent in the culture medium. Further, there was no cellular uptake in the KB cells when folic acid was added in the culture medium (Fig. 3B, E). This result directly indicates that the PEG-folate was taken up by a FR-mediated endocytosis process, in accordance with the FACS results.
In the result of the ITLC-SG chromatographic strips, with saline as the mobile phase, labeling efficiency was shown approximately above 98% and the stability of radiolabeled conjugates was at least 95% until 6 hr in saline (Fig. 4A, B). Therefore, radiolabeled PEG-folate is very stable until 6 hr. Also, the result of the stability test in human serum showed a 90.6% yield until 16 hr (Fig. 4C).
In order to determine whether the cellular uptake of radiolabeled conjugate depends on the folate receptor, KB cells and A549 cells were treated with radiolabeled PEG-folate and competition studies were carried out for the KB cells with using radiolabeled PEG and PEG-folate added folic acid. The result was that the KB cells showed higher levels of the 99mTc CPM of PEG-folate than did the A549 cells (Fig. 5A). The 99mTc CPM of the PEG or the PEG-folate added folic acid (Fig. 5B) showed lower levels than did the 99mTc CPM of PEG-folate in the KB cells. Especially, the cellular uptake between PEG-folate and PEG-folate with folic acid showed a significant difference.
The gamma camera image of 99mTc PEG-folate (11 MBq/300 µL) via tail vein injection demonstrated receptor mediated uptake. After administration of 99mTc PEG-folate, the images were recorded at 30 min, 1, 2, 3 and 4 hr. Until 4 hr, the images especially showed specific binding to the tumor and strong uptake in comparison with normal muscle (Fig. 6). Moreover, the tumor-to-normal muscle ratio reached 4.3 at 4 hr (Fig. 7). In the competition study, the tumor uptake was blocked by the co-injection of free folic acid, and the image indicates our conjugate is not taken up via the folate receptor for tumor localization, and the tumor to normal muscle ratio was under 1.2 at 4 hr.
We radiolabled PEG-folate with 99mTc to target the FRs with relative stability, and this was able to localize and detect the regions with FR overexpression. The results of this study demonstrated that 99mTc PEG-folate was taken up by a FR-mediated endocytosis process. Most of the current studies about folate have been conducted for drug delivery systems (DDSs), and few studies have shown possibility of PEG-folate as an imaging agent for diagnostic imaging (13-16). The significance of this study is that we proved the targetability of PEG-folate conjugates to FRs on cells through in vitro and in vivo studies with using bifunctional PEG.
To synthesize folate with a PEG chain, the α- and γ-carboxylic groups at the end of folic acid should be activated by DCC/NHS chemistry. However, it is known that the γ-carboxylic acid was primarily conjugated due to its higher reactivity. When activated folic acid is conjugated with PEG, it becomes hydrophilic and the circulation time is increased by PEG. The circulation time depends on the molecular weight of PEG, and it is well known that if a wide range of targeting moieties were conjugated directly to the distal end of the PEG chain, the circulation time is increased. From the standpoint of targeting, it is highly desirable to directly conjugate a targeting ligand to the PEG end group, which would facilitate receptor-mediated endocytosis of the PEG modified complexes (17-20). For these reasons, synthesis using folate and PEG has the possibility to be applied to nuclear imaging of the tumor biology of FR positive tumor cells and for following-up the therapeutic effect and prognosis.
The result of fluorescence microscopy of the KB cells clearly indicated that the uptake of PEG-folate significantly increased in the folate free medium compared with that of the A549 cells, and the competition with free folic acid blocked the cell uptake of PEG-folate in the KB cells. These facts can be confirmed by the results of the cellular uptake of radiolabeled conjugates. These results confirmed the PEG-folate entered into the KB cells through the folate receptor endocytosis pathway. Folic acid is taken up via the FR on the cell membrane and it acts as a precursor of tetrahydrofolate in the nucleus (21). The result of strong uptake of the cellular nucleus in this study demonstrates that the PEG-folate conjugate moves through the nuclear membrane (Fig. 3).
Functional groups such as -COOH, -OH, -NH2, and -SH can form coordinate covalent bonds with 99mTc (22, 23). Generally, PEG can't be radiolabeled with 99mTc without using a chelator. In this study, we infer that 99mTc forms coordinate covalent bonds with the amine group of PEG (Jeffamine), which specifically serves as a chelating moiety.
Studies related to folate as an imaging agent have used EC20, MAG3 and DTPA for chelation with 99mTc, and the folate conjugate using PEG was also labeled with 99mTc using DTPA (24-26). Yet we directly labeled the PEG-folate with 99mTc, and the yield of the radiolabeled conjugate was over 95% until 6 hr in vitro and it was 90% until 6 hr in serum conditions. The formation of a coordinate covalent bond between 99mTc and the amine group at the end of PEG, and the relatively large molecular weight and flexibility of PEG are considered the characteristics that contributed to stable labeling efficiency.
On the in vivo study, we succeeded in performing tumor targeted nuclear imaging using 99mTc PEG-folate in mice, and the tumor-to-normal muscle ratio was approximately 4. On the competitive study, binding of 99mTc PEG-folate was blocked by the co-injection of free folic acid, so the images didn't show uptake in tumor region and the tumor to normal muscle ratio was approximately 1. These results mean that uptake of PEG-folate was inhibited by folic acid and our conjugate specifically binds to FR and this appropriates to the results of the cellular uptake studies.
In summary, we were able to stably label PEG-folate with 99mTc and we confirmed that our conjugate specifically binds to FR. On the results of flow cytometric analysis, fluorescence microscopy study and measurement of 99mTc CPM, we confirmed that PEG-folate was endocytosed via the FR in vitro. Especially, the CPM of 99mTc PEG-folate was much higher than that of 99mTc PEG, and the variation was almost 60%. Moreover, we applied our conjugates in vivo, and the results of the images were well accorded with the results of the cellular uptake experiments. In conclusion, we could specifically trace the FR on the cell surface with using the PEG-folate conjugates in the in vitro and in vivo studies.
Figures and Tables
Fig. 2
Flow cytometric analysis of the KB (A) cells and A549 (B) cells treated with PEG-FITC (thick black line), PEG-folate-FITC (thin black line), and PEG-folate-FITC added folic acid (gray) in the culture media without folic acid.
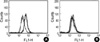
Fig. 3
Fluorescent microscopic pictures of KB cells with folate-PEG-FITC when folic acid was absent (A, D), and present in the culture media (B, E) and the A549 cells with folate-PEG-FITC when folic acid was absent (C, F).
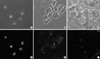
Fig. 4
ITLC chromatography of 99mTc PEG-folate (A), labeling efficiency of 99mTc PEG-folate (B), and the stability of 99mTc PEG-folate in human serum (C).
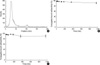
Fig. 5
Cellular uptake of radiolabeled PEG-folate in KB and A549 cells (A) and the study on contrast using PEG, PEG-folate and PEGfolate with folic acid in the KB cells (B). Data represent the means of three independent experiments±SD (*, p<0.05).
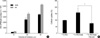
References
1. Antony AC. Folate receptors: reflections on a personal odyssey and a perspective on unfolding truth. Adv Drug Deliv Rev. 2004. 56:1059–1066.


2. Kwon GS, Okano T. Polymeric micelle as new drug carriers. Adv Drug Deliv Rev. 1996. 16:107–116.
3. Yoo HS, Oh JE, Lee KH, Park TG. Biodegradable nanoparticles containing doxorubicin--PLGA conjugates for sustained release. Pharm Res. 1999. 16:1114–1118.
4. Lee RJ, Low PS. Delivery of liposomes into cultured KB cells via folate receptor-mediated endocytosis. J Biol Chem. 1994. 269:3198–3204.


5. Toffoli G, Claudia C, Russo A, Gallo A, Bagnoli M, Boiocchi M. Overexpression of folate binding protein in ovarian cancers. Int J Cancer. 1997. 74:193–197.


6. Sudimack J, Lee RJ. Targeted drug delivery via the folate receptor. Adv Drug Deliv Rev. 2000. 41:147–162.


7. Cho KC, Kim SH, Jeong JH, Park TG. Folate receptor-mediated gene delivery using folate-poly(ethylene glycol)-poly(L-lysine) conjugate. Macromol Biosci. 2005. 5:512–519.


8. Liu M, Xu W, Xu LJ, Zhong GR, Chen SL, Lu WY. Synthesis and biological evaluation of diethylenetriamine pentaacetic acid-polyethylene glycol-folate: a new olate-derived, 99mTc-based radiopharmaceutical. Bioconjugate Chem. 2005. 16:1126–1132.
9. Yoo HS, Park TG. Folate receptor targeted biodegradable polymeric doxorubicin micelles. J Control Release. 2004. 96:273–283.


10. Mathias CJ, Wang S, Lee RJ, Waters DJ, Low PS, Green MA. Tumor-selective radiopharmaceutical targeting via receptor-mediated endocytosis of gallium-67-deferoxamine-folate. J Nucl Med. 1996. 37:1003–1008.
11. Mathias CJ, Wang S, Waters DK, Turek JJ, Low PS, Green MA. Indium-111-DTPA-folate as a potential folate-receptor-targeted radiopharmaceutical. J Nucl Med. 1998. 39:1579–1585.
12. Mathias CJ, Wang S, Low PS, Waters DJ, Green MA. Receptor mediated targeting of 67Ga-deferoxamine-folate to folate-receptor positive human KB tumor xenografts. Nucl Med Biol. 1999. 26:23–25.
13. Yoo HS, Park TG. Folate-receptor-targeted delivery of doxorubicin nano-aggregates stabilized by doxorubicin--PEG--folate conjugate. J Control Release. 2004. 100:247–256.


14. Trump DP, Mathias CJ, Yang Z, Low PS, Marmion M, Green MA. Synthesis and evaluation of 99mTc (CO)(3)-DTPA-folate as a folate-receptor-targeted radiopharmaceutical. Nucl Med Biol. 2002. 5:569–573.
15. Zhang L, Houb S, Maob S, Wei D, Song X, Lu Y. Uptake of folate-conjugated albumin nanoparticles to the SKOV3 cells. Int J Pharm. 2004. 287:155–162.


16. Gabizon A, Shmeeda H, Horowitz AT, Zalipsky S. Tumor cell targeting of liposome-entrapped drugs with phospholipid-anchored folic acid--PEG conjugates. Adv Drug Deliv Rev. 2004. 56:1177–1192.


17. Sabharanjak S, Mayor S. Folate receptor endocytosis and trafficking. Adv Drug Deliv Rev. 2004. 56:1099–1109.


18. Shi G, Guo W, Stephenson SM, Lee RJ. Efficient intracellular drug and gene delivery using folate receptor-targeted pH-sensitive liposomes composed of cationic/anionic lipid combinations. J Control Release. 2002. 80:309–319.


19. Benns JM, Mahato RI, Kim SW. Optimization of factors influencing the transfection efficiency of folate-PEG-folate-graft-polyethylenimine. J Control Release. 2002. 79:255–269.


20. Wang S, Low PS. Folate-mediated targeting of antineoplastic drugs, imaging agents, and nucleic acids to cancer cells. J Control Release. 1998. 53:39–48.


21. Wong JY, Kuhl TL, Israelachvili JN, Mullah N, Zalipsky S. Direct measurement of a tethered ligand--receptor. Science. 1997. 275:820–822.
22. Saha GB. Radiopharmaceuticals and Method of Radiolabeling. Fundamentals of Nuclear Pharmacy. 1998. 4th edtion. New York: Springer-Verlag;80–111.
24. Okarvi SM, Jammaz IA. Preparation and in vitro and in vivo evaluation of technetium-99m-labeled folate and methotrexate conjugates as tumor imaging agents. Cancer Biother Radiopharm. 2006. 21:49–60.


25. Guo WJ, Hinkle GH, Lee RJ. 99mTc-HYNIC-folate: a novel receptor-based targeted radiopharmaceutical for tumor imaging. J Nucl Med. 1999. 40:1563–1569.
26. Reddy JA, Xu LC, Parker N, Vetzel M, Leamon CP. Preclinical evaluation of (99m)Tc-EC20 for imaging folate receptor-positive tumors. J Nucl Med. 2004. 45:857–866.