Abstract
Dioscorea species continue to be used in traditional Chinese medicine, and represent a major source of steroid precursors for conventional medicine. In the previous study, We isolated glycoprotein (GDB) from Dioscorea batatas, characterized, and demonstrated immunostimulating activity in C57BL/6 mice. The aim of this study was to investigate the mechanism whereby GDB activates macrophages. Macrophages activation by GDB was investigated by analyzing the effects of GDB on nitric oxide (NO) production, iNOS expression, mitogen activated protein kinase (MAPK) phosphorylation, and transcription factor activation. In the presence of IFN-γ, GDB strongly stimulated macrophages to express iNOS and produce NO. Furthermore, the activation of p38 was synergistically induced by GDB plus IFN-γ , but SB203580 (a p38 inhibitor) inhibited GDB plus IFN-γ-induced p38 activation. This study indicates that GDB is an important activator of macrophages. Furthermore, due to the critical role that macrophage activation plays in innate immune response, the activation effects of GDB on macrophages suggest that GDB may be a useful immunopotentiating agent.
Dioscorea species are used in conventional medicine as a major source of steroid precursors, and are also used in traditional Chinese medicine [1]. These plants have immunomodulatory activity in vitro on the viability, cell-mediated cytotoxicity, and on IFN-γ secretion by splenic lymphocytes [2]. Furthermore, phytochemical investigations have shown that steroid saponins are the active principles of Dioscorea species [1]. Members of this species have long been used in traditional medicine to treat poor appetite, chronic diarrhea, asthma, dry coughs, frequent or uncontrollable urination, diabetes, and emotional instability [1]. Plants of the genus Dioscorea of the Dioscoreaceae family are commonly referred to as yams. Immunostimulating activities of yam storage protein from Dioscorea alata, japonica and batatas have been described previously [3-5]. Yam is an important crop, which is basically made up of carbohydrates but also constitutes an important source of proteins accounting for 1~3% of the fresh tubers [6]. We isolated glycoprotein from Dioscorea batatas, characterized, and demonstrated immunostimulating activity in C57BL/6 mice [7].
Macrophages can be activated by cytokines, such as, IFN-γ, or by microbial components, such as, LPS [8,9], and play pivotal roles in both nonspecific and specific immunity. For nonspecific immunity, macrophages trap and kill microbes, and release inflammatory mediators, such as, nitric oxide (NO). For nonspecific immunity, macrophages act as antigen presenting cells and release cytokines, such as, tumor necrosis factor (TNF-α), IL-1, IL-6, and IL-12 to regulate helper T cells [10]. Furthermore, activated macrophages suppress the growths of a variety of tumor cells and microorganisms [11]. However, strong activation of macrophages causes the releases excessive cytokines and mediators, including NO, which has been implicated in many pathophysiological conditions including inflammation, atherosclerosis, and septic shock [12]. Due to the critical role that macrophage activation plays in innate immune response, immunomodulating compounds target macrophages [13-15].
Macrophage activation by certain external stimuli including lipopolysaccharide, a component of the gram-negative bacterial cell wall, results in the phosphorylation of mitogen activated protein kinase (MAPK) family, which includes ERK1/2, stress-activated protein kinases (SAPK)/c-Jun NH2-terminal kinase (JNK), and p38 MAPK [16]. p38, ERK1/2, and SAPK/JNK are serine threonine kinases that are located in the cytoplasm until activated by dual phosphorylation of their Tyr and Thr residues at Thr-Gly-Tyr, Thr-Glu-Tyr, or Thr-Pro-Tyr, respectively [17]. The activation of MAPK stimulates transcription factors, such as, NF-κB and AP-1 [18,19].
In the present study, we investigated the synergistic effect of glycoprotein (GDB) isolated from Dioscorea batatas and IFN-γ on iNOS gene expression by macrophages. We further analyzed the effects of GDB on the activation of MAPK in RAW264.7cells.
Male C57BL/6 mice weighing 21~23 g were housed under a 12-h light/dark cycle in a temperature-controlled room (22~24℃). Mice were given access to standard chow and water ad libitum.
The tubers of Dioscorea batatas were harvested in May at the Okcheon County in North Chungcheong Province, South Korea, and were stored at 4℃ until used. For isolation of the proteins, whole tubers were used. Glycoproteins were isolated from Dioscorea batatas according to the procedure of previously reported [7,20]. In brief, tubers were peeled and homogenized and subjected to hydrophobic chromatography on a phenyl-Toyopearl 650 M column (Tosoh, Japan) equilibrated with 50 mM Tris-HCl buffer (pH 7.0) containing ammonium sulfate to give 25% saturation. Elution was performed by a decrease of ammonium sulfate concentration from 25 to 0% in 50 mM Tris-HCl buffer (pH 7.0). The fractions yielding absorbance at 280 nm were pooled, dialyzed against distilled water, and lyophilized in a freeze dryer. The lyophilized proteins were subjected to an anion-exchange chromatography on a HiTrap Q HP column (GE Healthcare).
RAW 264.7 cells (ATCC TIB71) were grown in RPMI 1640 supplemented with 10% fetal bovine serum, 2 mM L-glutamine, 100 U/ml penicillin and 100 µg/ml streptomycin. Cells were then cultured in the presence of 5% CO2 at 37℃.
RAW 264.7 cells were stimulated with GDB plus FN-γ for 24 h. Culture supernatants were collected and the accumulation of NO2- in culture supernatants was as an indicator of NO production in the medium as previously described [21]. The isolated supernatants were mixed with an equal volume of Griess reagent (1% sulfanilamide, 0.1% naphthylethylenediamine dihydrochloride, and 2% phosphoric acid) and incubated at room temperature for 10 min. Using NaNO2 to generate a standard curve, nitrite production was measured by an O.D. reading at 550 nm.
Total RNA was isolated with Tri Reagent (Molecular Researh Center, Cincinnati, OH, USA). The forward and reverse primer sequences are as follows: iNOS: 5'-CTG CAG CAC TTG GAT CAG GAA CCT G-3', 5'-GGG AGT AGC CTG TGT GCA CCT GGA A-3'; and β-actin: 5'-TGG AAT CCT GTG GCA TCC ATG AAA C-3', 5'-TAA AAC GCA GCT CAG TAA CAG TCC G-3'. Equal amounts of RNA were reverse transcribed into cDNA with oligo(dT)15 primers. PCR was performed with cDNA and each primer. Samples were heated to 94℃ for 5 min and cycled 30 times at 94℃ for 1 min, 55℃ for 1.5 min, and 94℃ for 1 min, after which an additional extension step at 72℃ for 5 min was included. PCR products were electrophoresed in 8% polyacrylamide gel followed by staining in ethidium bromide. The iNOS and β-actin primers produce amplified products at 311 and 349 bp, respectively.
Whole cell lysates were separated by 10% SDS-PAGE, then electro-transferred to nitrocellulose membranes (Amersham International, Buckinghamshire, UK). The membranes were preincubated for 1 hr at room temperature in Tris-buffered saline, pH 7.6 containing 0.05% Tween-20 and 3% bovine serum albumin. The nitrocellulose membranes were incubated with specific antibodies. Immunoreactive bands were then detected by incubation with conjugates of antirabbit IgG with horseradish peroxidase and enhanced chemiluminescence reagents (Amersham). Antibodies against, p38, phospho-p38 (Thr180/Tyr182), ERK1/2, phospho-ERK1/2 (Thr202/Tyr204), JNK, phospho-SAPK/JNK (Thr183/Tyr185), and β-actin were obtained from Cell Signaling Biotechnology, Inc (MA, USA). Goat anti rabbit IgG-HRP antibody was from purchased from Santa Cruz Biotechnology, Inc (CA, USA).
RAW264.7 cells were cultured on slide glass and treated with GDB (25 µg/ml) and IFN-γ (10 ng/ml) for 24 h. Morphological change and iNOS expression were analyzed using immunofluorescence staining. The slide was fixed with 4% paraformaldehyde for 10 min and washed with 0.01 M phosphate buffered saline (PBS, pH 7.4). The sample was blocked with 3% BSA in 1X PBS/0.03% Triton X-100 in a humidified chamber for 1 h at room temperature and incubated with rabbit anti-iNOS (1:200 dilutions in 1X PBS/0.03% Triton X-100) at 4℃ in a humidified chamber overnight. The slide was washed, hybridized with secondary antibodies conjugated with fluorescein isothiocyanate (FITC) for 1 h at room temperature in the dark. The slide was then washed and mounted with GEL/MOUNT solution containing anti-fading agents and was observed by laser scanning confocal microscopy (FV300, Olympus, Japan).
RAW 264.7 cells were grown at 5×105 cells/ml in petri-dish and then treated with GDB for 2 h. Nuclear extracts were prepared as earlier described [22]. Briefly, cells were lysed with hypotonic buffer (10 mM HEPES, 1.5 mM MgCl2, pH 7.5) and nuclei were pelleted by centrifugation at 3,000×g for 5 min. Nuclear lysis was performed using a hypertonic buffer (30 mM HEPES, 1.5 mM MgCl2, 450 mM KCl, 0.3 mM EDTA, 10% glycerol, 1 mM DTT, 1 mM PMSF, 1 µg/ml of aprotinin, and 1 µg/ml of leupeptin). Following lysis, the samples were centrifuged at 14,500×g for 15 min, and supernatant was retained for use in the DNA binding assay. The double-stranded oligonucleotides were end-labeled with [γ-32P]-ATP. Nuclear extracts (5 µg) were incubated with poly (dI-dC) and the [32P]-labeled DNA probe in binding buffer (100 mM KCl, 30 mM HEPES, 1.5 mM MgCl2, 0.3 mM EDTA, 10% glycerol, 1 mM DTT, 1 mM PMSF, 1 µg/ml of aprotinin, and 1 µg/ml of leupeptin) for 10 min. DNA binding activity was separated from free probe using a 5% polyacrylamide gel in 0.5X TBE buffer. Following electrophoresis, the gel was dried and subjected to autoradiography.
The mean±S.D. was determined for each treatment group in a given experiment. When significant differences occurred, treatment groups were compared to the vehicle control using a Dunnett's two-tailed t test [23].
The glycoproteins (GDB) isolated from Dioscorea batatas were analyzed by SDS-PAGE and produced a single band at 30 kD (Fig. 1A). To investigate macrophage activation by GDB, we treated RAW264.7 macrophage cells with GDB (50 µg/ml) in the presence of IFN-γ (10 ng/ml) and analyzed iNOS expression by immunofluorescence staining (Fig. 1B). GDB plus IFN-γ strongly stimulated macrophages to produce iNOS and induced morphological changes. To analyze the effect of GDB plus IFN-γ on iNOS gene expression further, we performed Western blot analysis and RT-PCR. GDB plus IFN-γ significantly induced iNOS gene expression, whereas GDB plus IFN-γ alone did not (Fig. 1C, D).
To investigate macrophage activation by GDB, we analyzed the effects of GDB on nitrite levels, which is produced from NO in RAW264.7 cells. RAW264.7 cells were treated with IFN-γ (1, 2.5, 5, and 10 ng/ml) in the presence or absence of GDB (25 µg/ml) for 24 h. Supernatants were then isolated and analyzed for nitrite generation. GDB (25 µg/ml) plus IFN-γ (1, 2.5, 5, and 10 ng/ml) significantly induced nitrite generation in a dose-dependent manner, whereas IFN-γ alone did not (Fig. 2A). LPS (200 ng/ml) was used as a positive control. The synergistic induction of nitrite by GDB (1, 10, 25, and 50 µg/ml) plus IFN-γ (10 ng/ml) was further confirmed in RAW264.7 cells (Fig. 2B). GDB (1, 10, 25, and 50 µg/ml) alone did not increase the nitrite generation.
Most cellular responses to extracellular stimuli are mediated by kinase and phosphatase cascades. The MAPKs are one of the most important kinase families in inflammatory cells, and its members have been strongly conserved through evolution, suggesting that they play vital roles in intracellular signaling. Thus, since MAPKs play important roles in macrophage activation, we analyzed the effects of GDB on MAPK activity by immunoblotting. When cells were treated with GDB (1, 10, and 25 µg/ml) for 30 min, we found that phosphorylation of ERK1/2, and especially of SAPK/JNK, was markedly induced (Fig. 3A). ERK1/2 and SAPK/JNK phosphorylations were induced by IFN-γ (10 ng/ml), and the phosphorylation was not further activated by GDB. The phosphorylation of p38 kinase was not strongly induced by GDB or IFN-γ. However, interestingly cotreatment of RAW264.7 cells with GDB and IFN-γ strongly induced p38 phosphorylation (Fig. 3). This synergistic induction of p38 by GDB and IFN-γ corresponds well with the activation of iNOS gene expression. These results suggest that GDB activates macrophages by activating signal transduction pathways, such as, p38, ERK1/2, and SAPK/JNK.
We further investigated whether the p38 kinase pathway is involved in GDB and IFN-γ-induced nitrite generation. We specifically blocked the p38 kinase pathway and then monitored nitrite production when RAW 264.7 cells were challenged with GDB plus IFN-γ. SB203580, a bicyclic imidazole inhibitor of p38, inhibited GDB plus IFN-γ-induced nitrite production (Fig. 4A). However, PD98059, a specific inhibitor of MEK-1, did not. LPS-induced nitrite production was also inhibited by SB203580 but not by PD98059 (Fig. 4A). However, GDB plus IFN-γ-induced nitrite production was not inhibited by polymyxin B (an LPS inhibitor), whereas LPS-induced nitrite generation was strongly inhibited by polymyxin B (Fig. 4B). These results suggest that p38 kinase pathway is importantly involved in the regulation of iNOS expression induced by GDB plus IFN-γ.
To investigate the mechanism whereby GDB plus IFN-γ activate macrophages, we analyzed the effect of GDB on NF-κB, AP-1, and Oct 1, whose binding sites are located in the promoter of iNOS gene. EMSA showed that treating RAW264.7 cells with GDB plus IFN-γ for 2 h did induce NF-κB DNA binding activity, whereas GDB alone dose-dependently induced NF-κB DNA binding activity (Fig. 5A). Both AP-1 and Oct1 were found to have small basal DNA binding activities and to be moderately induced by GDB (Fig. 5B, C). The specificity of bindings was demonstrated by competition assays using 32P-unlabeled κB or mutant κB probes (data not shown). These results suggest that GDB activates NF-κB, which is known to play an important role in the activation and production of cytokines in macrophages.
The present study demonstrates that GDB and IFN-γ synergistically induce NO production. In the presence of IFN-γ, GDB strongly increased NO production, whereas GDB alone did not activate macrophages to produce NO (Fig. 2). The synergistic induction of iNOS gene expression by GDB plus IFN-γ was further confirmed by Western blotting and RT-PCR (Fig. 1C, D). Although activated macrophages suppress the growths of a variety of tumor cells and microorganisms [11], excessive production of NO is implicated in many pathophysiological conditions. For example LPS, a strong inducer of NO, cause cell and tissue damage, which can lead to multiple organ failure - the clinical hallmark of sepsis [12]. Synergism with IFN-γ (an endogenous mediator of immune response) highlights the importance of the immune modulatory role of GDB.
We also found that GDB activated MAPKs. Interestingly, GDB plus IFN-γ synergistically activated p38 phosphorylation. Furthermore, this synergistic activation of p38 by GDB plus IFN-γ corresponded well with the synergistic induction of NO generation and iNOS expression. In the presence of IFN-γ, GDB strongly increased p38 phosphorylation, whereas GDB alone did not activate macrophages to phosphorylate p38 (Fig. 3). We also confirmed the involvement of the p38 pathway in GDB plus IFN-γ mediated NO production, because NO generation was dramatically diminished in the presence of SB203580 (a specific inhibitor of p38 MAPK). In contrast PD98059 (a specific inhibitor of MEK1) had no effect on NO generation by GDB plus IFN-γ. The p38 kinase pathway is also important for LPS-induced NO production, as shown in Fig. 4A. In a previous study [24], we confirmed that the p38 pathway is specifically involved in LPS-induced iNOS expression.
Although GDB plus IFN-γ was not found to synergistically activate SAPK/JNK, GDB alone strongly and dose-dependently induced SAPK/JNK phosphorylation (Fig. 3). These results suggest that GDB mediates immune modulation via the differential activations of MAPKs by itself or in combination with other cytokines. MAPKs transmit extracellular signals to the nucleus, where the transcriptions of specific genes are induced by the phosphorylations and activations of transcription factors. Furthermore, different environmental stimuli trigger the activations of different MAPKs via different pathways. For example, the ERK MAPKs are activated by a Ras-dependent pathway in response to many growth factors and hormones [25], whereas the SAPK/JNK and p38 MAPKs are activated by environmental stresses, such as, UV radiation, osmotic shock, heat shock, protein synthesis inhibitors, LPS, and by proinflammatory cytokines, such as, IL-1 and TNF [19,26].
Macrophages play a pivotal role in immune response by presenting antigens to lymphocytes during the development of specific immunity and by releasing soluble factors [27]. Macrophages also produce protective factors that are involved in host defense and inflammation. Once activated, macrophages produce a number of cytotoxic molecules, such as, NO [9], which is also cytotoxic to certain tumor cells [28]. In a previous study, we found that GDB can exert significant immunomodulatory effects on specific immune responses mediated by macrophages, lymphocytes, and NK cells in vitro and in vivo [7]. GDB treatment of C57BL/6 mice caused a significant increase in the numbers of lymphocytes and macrophages in the peritoneal cavity, and both of these cell types play important roles in the first line of immunological defense against tumor cells. Therefore, we assessed the effects of GDB on NK cells and macrophage function. We found that NK cell activity and the cytotoxic effects of macrophages on tumor cells significantly increased in GDB-treated groups.
Although we could not identify the membrane receptor for GDB in the present study, some membrane proteins, such as, CR3, CD14, and Toll-like receptors (TLRs), are known to act as receptors in macrophages. Complement receptor CR3 (also called CD11b/CD18, Mac-1, and αMβ2-integrin) is a membrane receptor for β-glucans [29], and CD14 is a glycosylphosphatidylinositol-anchored protein expressed on the surfaces of monocytes and neutrophils that binds LPS with high affinity [30]. These receptors expressed on the surfaces of neutrophils, monocytes, macrophages, and NK cells and are important in numerous cell-cell and cell-substrate interactions [31]. The TLRs are a mammalian transmembrane protein family and are known to play important roles in innate immune recognition [32].
The immunostimulating activities of yam storage protein from Dioscorea alata and japonica have been previously described [3,4]. Yam is basically composed of carbohydrates but also contains many proteins, which account for 1~3% of the weight of fresh tubers [6]. Two proteins identified in D. alata tubers, dioscorins and phytoglycoproteins [33,34] are known to activate MAP kinases (ERK, p38, and JNK) and NF-κB via the TLR-4 signal transduction pathway and increases the expressions of pro-inflammatory cytokines, such as, TNF-α, IL-1β, and IL-6, in several cells including RAW 264.7 macrophages, murine monocytes, and human monocytes [4,35]. We previously isolated glycoprotein from Dioscorea batatas, and characterized and demonstrated its immunostimulatory activity [7].
In summary, the present study shows that GDB is a potent modulator of immune response. Based on our findings, macrophage activation best explains this biological effect. Due to the critical role that macrophage activation plays in innate immune response, the activation of macrophages by GDB suggests that this compound should be regarded as a potentially useful immunopotentiating agent.
References
1. Chen H, Wang C, Chang CT, Wang T. Effects of Taiwanese yam (Dioscorea japonica Thunb var. pseudojaponica Yamamoto) on upper gut function and lipid metabolism in Balb/c mice. Nutrition. 2003; 19:646–651. PMID: 12831952.


2. Jin UH, Kim DI, Lee TK, Lee DN, Kim JK, Lee IS, Kim CH. Herbal formulation, Yukmi-jihang-tang-Jahage, regulates bone resorption by inhibition of phosphorylation mediated by tyrosine kinase Src and cyclooxygenase expression. J Ethnopharmacol. 2006; 106:333–343. PMID: 16513308.


3. Lin PL, Lin KW, Weng CF, Lin KC. Yam storage protein dioscorins from Dioscorea alata and Dioscorea japonica exhibit distinct immunomodulatory activities in mice. J Agric Food Chem. 2009; 57:4606–4613. PMID: 19378946.


4. Fu SL, Hsu YH, Lee PY, Hou WC, Hung LC, Lin CH, Chen CM, Huang YJ. Dioscorin isolated from Dioscorea alata activates TLR4-signaling pathways and induces cytokine expression in macrophages. Biochem Biophys Res Commun. 2006; 339:137–144. PMID: 16297883.


5. Choi EM, Koo SJ, Hwang JK. Immune cell stimulating activity of mucopolysaccharide isolated from yam (Dioscorea batatas). J Ethnopharmacol. 2004; 91:1–6. PMID: 15036459.


7. Huong PT, Lee CH, Li MH, Lee MY, Kim JK, Lee SM, Seon JH, Lee DC, Jeon YJ. Characterization and immunopotentiating effects of the glycoprotein isolated from dioscorea batatas. Korean J Physiol Pharmacol. 2011; 15:101–106. PMID: 21660150.


8. Higuchi M, Higashi N, Taki H, Osawa T. Cytolytic mechanisms of activated macrophages. Tumor necrosis factor and L-arginine-dependent mechanisms act synergistically as the major cytolytic mechanisms of activated macrophages. J Immunol. 1990; 144:1425–1431. PMID: 2303713.
9. Stuehr DJ, Nathan CF. Nitric oxide. A macrophage product responsible for cytostasis and respiratory inhibition in tumor target cells. J Exp Med. 1989; 169:1543–1555. PMID: 2497225.


10. Billack B. Macrophage activation: role of toll-like receptors, nitric oxide, and nuclear factor kappa B. Am J Pharm Educ. 2006; 70:102. PMID: 17149431.


11. Lowenstein CJ, Alley EW, Raval P, Snowman AM, Snyder SH, Russell SW, Murphy WJ. Macrophage nitric oxide synthase gene: two upstream regions mediate induction by interferon gamma and lipopolysaccharide. Proc Natl Acad Sci USA. 1993; 90:9730–9734. PMID: 7692452.


13. Jeon YJ, Han SB, Ahn KS, Kim HM. Differential activation of murine macrophages by angelan and LPS. Immunopharmacology. 2000; 49:275–284. PMID: 10996025.


14. Ban JY, Kim BS, Kim SC, Kim DH, Chung JH. Microarray analysis of gene expression profiles in response to treatment with melatonin in lipopolysaccharide activated RAW 264.7 cells. Korean J Physiol Pharmacol. 2011; 15:23–29. PMID: 21461237.


15. Li MH, Kothandan G, Cho SJ, Huong PT, Nan YH, Lee KY, Shin SY, Yea SS, Jeon YJ. Magnolol Inhibits LPS-induced NF-κB/Rel Activation by Blocking p38 Kinase in Murine Macrophages. Korean J Physiol Pharmacol. 2010; 14:353–358. PMID: 21311674.


16. Su B, Karin M. Mitogen-activated protein kinase cascades and regulation of gene expression. Curr Opin Immunol. 1996; 8:402–411. PMID: 8793994.


17. Raingeaud J, Gupta S, Rogers JS, Dickens M, Han J, Ulevitch RJ, Davis RJ. Pro-inflammatory cytokines and environmental stress cause p38 mitogen-activated protein kinase activation by dual phosphorylation on tyrosine and threonine. J Biol Chem. 1995; 270:7420–7426. PMID: 7535770.


18. Weinstein SL, Sanghera JS, Lemke K, DeFranco AL, Pelech SL. Bacterial lipopolysaccharide induces tyrosine phosphorylation and activation of mitogen-activated protein kinases in macrophages. J Biol Chem. 1992; 267:14955–14962. PMID: 1321821.


19. Whitmarsh AJ, Davis RJ. Transcription factor AP-1 regulation by mitogen-activated protein kinase signal transduction pathways. J Mol Med (Berl). 1996; 74:589–607. PMID: 8912180.


20. Gaidamashvili M, Ohizumi Y, Iijima S, Takayama T, Ogawa T, Muramoto K. Characterization of the yam tuber storage proteins from Dioscorea batatas exhibiting unique lectin activities. J Biol Chem. 2004; 279:26028–26035. PMID: 15047697.


21. Green LC, Wagner DA, Glogowski J, Skipper PL, Wishnok JS, Tannenbaum SR. Analysis of nitrate, nitrite, and [15N]nitrate in biological fluids. Anal Biochem. 1982; 126:131–138. PMID: 7181105.


22. Xie QW, Whisnant R, Nathan C. Promoter of the mouse gene encoding calcium-independent nitric oxide synthase confers inducibility by interferon gamma and bacterial lipopolysaccharide. J Exp Med. 1993; 177:1779–1784. PMID: 7684434.


23. Dunnett CW. A multiple comparison procedure for comparing several treatments with a control. J Am Statistics Assoc. 1995; 50:1096–1121.


24. Jeon YJ, Kim YK, Lee M, Park SM, Han SB, Kim HM. Radicicol suppresses expression of inducible nitric-oxide synthase by blocking p38 kinase and nuclear factor-kappaB/Rel in lipopolysaccharide-stimulated macrophages. J Pharmacol Exp Ther. 2000; 294:548–554. PMID: 10900231.
25. Robinson MJ, Cobb MH. Mitogen-activated protein kinase pathways. Curr Opin Cell Biol. 1997; 9:180–186. PMID: 9069255.


26. Ip YT, Davis RJ. Signal transduction by the c-Jun N-terminal kinase (JNK)--from inflammation to development. Curr Opin Cell Biol. 1998; 10:205–219. PMID: 9561845.
27. Adams DO, Hamilton TA. The cell biology of macrophage activation. Annu Rev Immunol. 1984; 2:283–318. PMID: 6100475.


28. Hibbs JB Jr, Taintor RR, Vavrin Z. Macrophage cytotoxicity: role for L-arginine deiminase and imino nitrogen oxidation to nitrite. Science. 1987; 235:473–476. PMID: 2432665.


29. Thornton BP, Větvicka V, Pitman M, Goldman RC, Ross GD. Analysis of the sugar specificity and molecular location of the beta-glucan-binding lectin site of complement receptor type 3 (CD11b/CD18). J Immunol. 1996; 156:1235–1246. PMID: 8558003.
30. Goyert SM, Ferrero E, Rettig WJ, Yenamandra AK, Obata F, Le Beau MM. The CD14 monocyte differentiation antigen maps to a region encoding growth factors and receptors. Science. 1988; 239:497–500. PMID: 2448876.


31. Hynes RO. Integrins: versatility, modulation, and signaling in cell adhesion. Cell. 1992; 69:11–25. PMID: 1555235.


32. Kopp EB, Medzhitov R. The Toll-receptor family and control of innate immunity. Curr Opin Immunol. 1999; 11:13–18. PMID: 10047546.


33. Oh PS, Lim KT. HeLa cells treated with phytoglycoprotein (150 kDa) were killed by activation of caspase 3 via inhibitory activities of NF-kappaB and AP-1. J Biomed Sci. 2007; 14:223–232. PMID: 17192825.
34. Lee SJ, Lim KT. Phytoglycoprotein inhibits interleukin-1beta and interleukin-6 via p38 mitogen-activated protein kinase in lipopolysaccharide-stimulated RAW 264.7 cells. Naunyn Schmiedebergs Arch Pharmacol. 2008; 377:45–54. PMID: 18204996.
35. Liu YW, Shang HF, Wang CK, Hsu FL, Hou WC. Immunomodulatory activity of dioscorin, the storage protein of yam (Dioscorea alata cv. Tainong No. 1) tuber. Food Chem Toxicol. 2007; 45:2312–2318. PMID: 17637490.


Fig. 1
Induction of iNOS expression by GDB plus IFN-γ. (A) GDB was isolated as described in "Methods". Isolated GDB was diluted 2- and 4- folds and analyzed by SDS-PAGE. (B) RAW264.7 cells were treated with GDB (25 µg/ml) and IFN-γ (10 ng/ml) for 24 h. Morphological change and iNOS expression were analyzed using immunofluorescence staining (upper column; with staining, lower column; without staining) and inverted microscope. (C) RAW264.7 cells were treated with GDB (1, 10, and 25 µg/ml) in the presence or absence of IFN-γ (10 ng/ml) for 8 h. Total RNA was then isolated and analyzed for iNOS using RT-PCR. (D) RAW264.7 cells were treated with GDB (1, 10, and 25 µg/ml) in the presence or absence of IFN-γ for 24 h. Cell lysates were then prepared and analyzed for the iNOS using Western blot analysis. One representative of three experiments is shown.
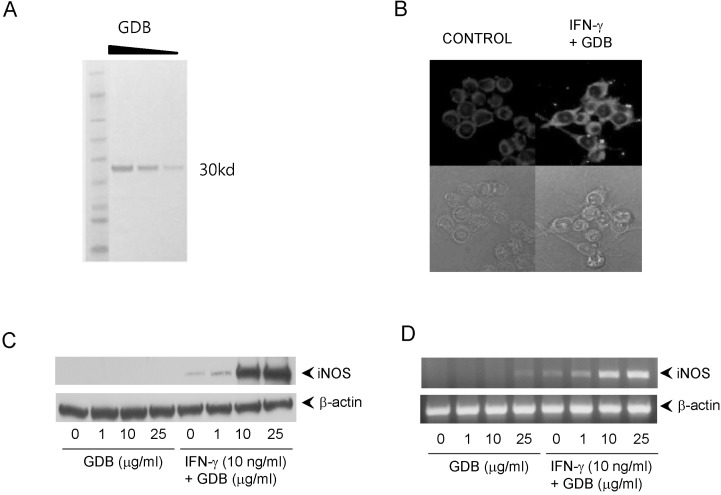
Fig. 2
Induction of NO production by GDB plus IFN-γ. (A) RAW264.7 cells were treated with IFN-γ (1, 2.5, 5, and 10 ng/ml) in the presence or absence of GDB (25 µg/ml) for 24 h. Supernatants were then isolated and analyzed for nitrite generation. LPS (200 ng/ml) was used as a positive control. (B) RAW264.7 cells were treated with GDB (1, 10, 25, and 50 µg/ml) in the presence or absence of IFN-γ (10 ng/ml) for 24 h. The results are presented as the mean±S.D. *Denotes significant differences (p<0.05) from the control group. One representative of three experiments is shown.
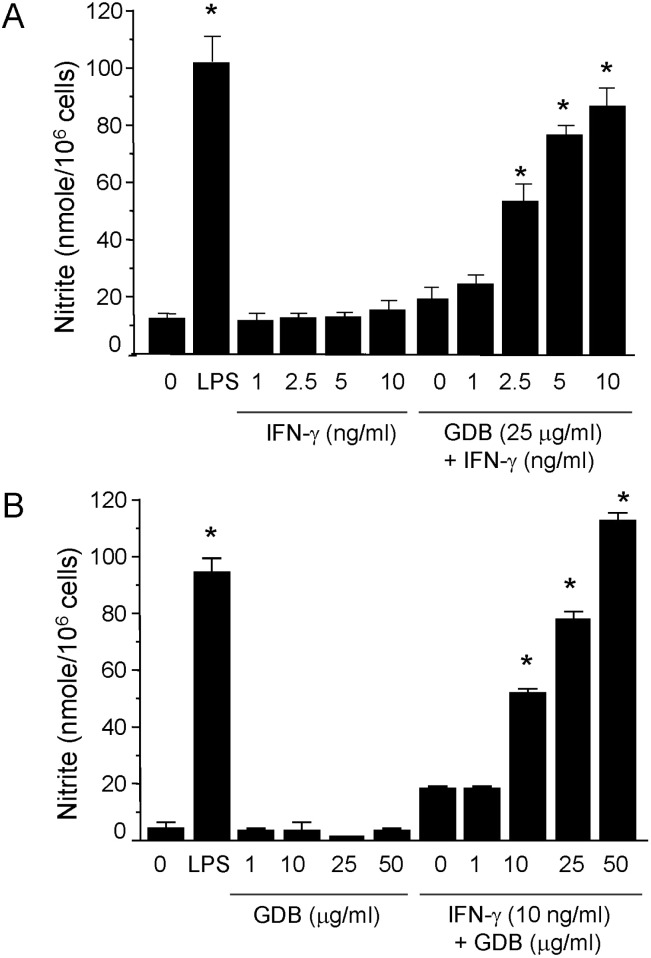
Fig. 3
Activation of p38 by GDB plus IFN-γ. (A) RAW264.7 cells were treated with GDB (1, 10, and 25 µg/ml) in the presence or absence of IFN-γ (10 ng/ml) for 30 min. Cell lysates were prepared and subjected to immunoblot assay using antibodies against phospho- and nonphospho-p38, ERK1/2 and SAPK/JNK proteins. (B) The density of p38 was analyzed by Image J program. β-actin was used as a control. One representative of three experiments is shown. *Denotes significant differences (p<0.05) from the control group.
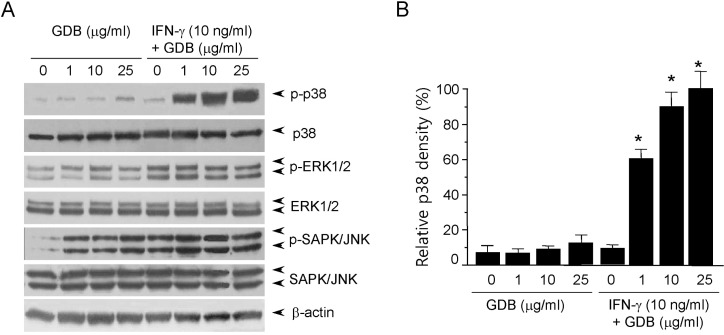
Fig. 4
Effect of SB203580 on iNOS expression in GDB plus IFN-γ-stimulated RAW 264.7 cells. (A) RAW 264.7 cells were pretreated with SB 203580 (30 µM) or PD98059 (50 µM) for 30 min before incubation with GDB (25 µg/ml) and IFN-γ (10 ng/ml) or LPS (200 ng/ml) for 24 h. (A) Supernatants were then isolated and analyzed for nitrite generation. (B) RAW264.7 cells were treated with Polymyxin B in the presence of GDB plus IFN-γ or LPS for 24 h. Supernatants were then isolated and analyzed for nitrite generation. One of two representative experiments is shown. *Denotes significant differences (p<0.05) from the control group.
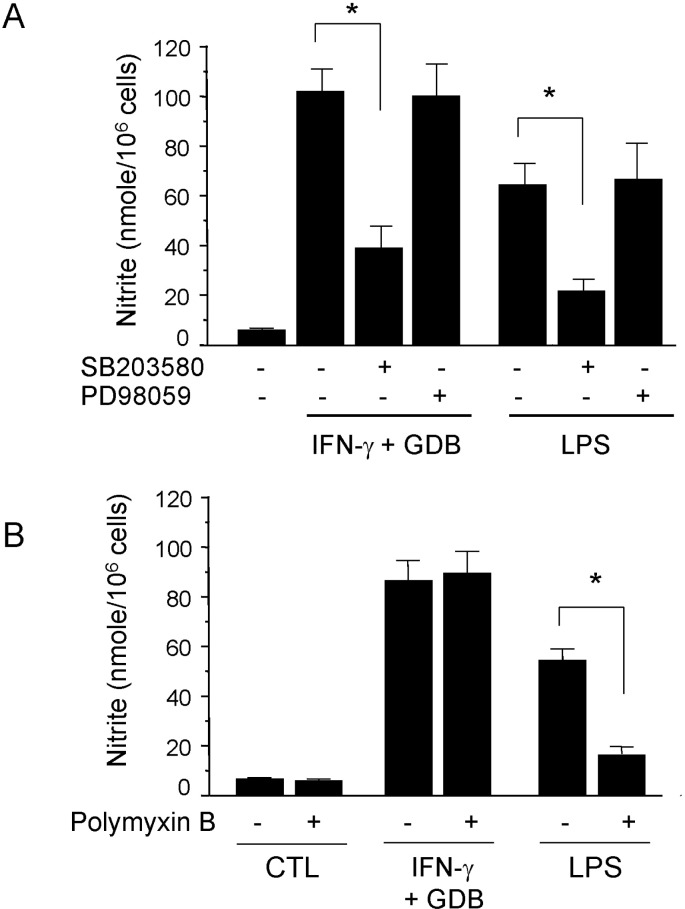
Fig. 5
Effects of GDB on the activation of NF-κB in RAW264.7 cells. RAW264.7 cells were treated with GDB (1, 10, and 25 µg/ml) in the presence or absence of IFN-γ (10 ng/ml) for 2 h. Nuclear extracts were isolated and analyzed for the determination of (A) NF-κB and (B) AP1 and Oct1. (C) using EMSA. One representative of three experiments is shown.
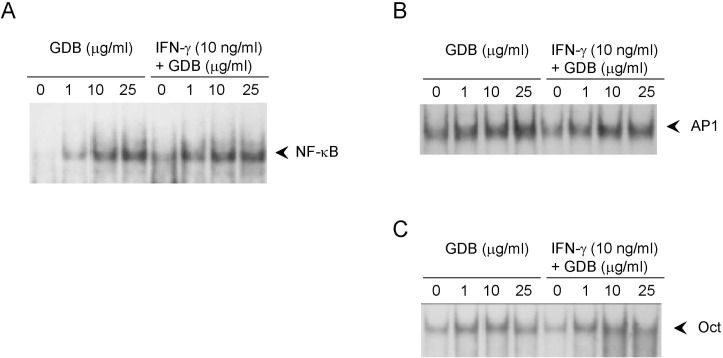