Abstract
Mitogen-activated protein kinases (MAPKs) play critical regulatory roles in the production of the pro-inflammatory cytokines and downstream signaling events which lead to inflammation. Inflammation is a primarily localized and protective response of host against microbial infection. Controlled inflammation is beneficial and necessary for host defense while uncontrolled inflammatory response results in inflammatory diseases such as septic shock, rheumatoid arthritis and cancer. The MAPK family consists of three subfamilies; the extracellular signal-regulated kinases (ERKs), the c-Jun N-terminal kinases (JNKs), and the p38 MAPKs. MAPKs are involved in transmitting extracellular signals to nucleus which leads to gene regulation. In this review, we summarize the current knowledge of ERK1/2, JNKs, and p38 MAPK members and their roles in inflammation.
The mammalian innate immune system acts like a guard and has ability to recognize microbial infection and plays its role in eradication of microbes. Inflammation is a primarily localized protective response by the host to eliminate destructive stimuli, and healing process for repairing injured tissue (1). Inflammation is characterized by five fundamental symptoms: redness, swelling, heat, pain, and loss of tissue function, which results in an increased permeability of the vascular endothelium as well as extravasations of immune cells (2). Macrophages and dendritic cells (DCs) are the central player in innate immunity; however, epithelial cells, endothelial cells, and fibroblasts also take part in innate immunity. Pathogen associated molecular patterns (PAMPs) are highly conserved among the microorganisms and germ line-encoded pattern recognition receptors (PRRs) are responsible for sensing of the microbes by these PAMPs (2, 3). Damaged cells releases endogenous molecules called as damage associated molecular patterns (DAMPs) which are also recognized by PRRs. The PRRs are divided into four different classes and called as Toll-like receptors (TLRs), C-type lectin receptors (CLRs), Retinoic acid inducible gene (RIG)-1-like receptors (RLRs) and NOD-like receptors (NLRs) (2~8).
Controlled inflammatory response is beneficial, as it provides protection against destructive stimuli while dysregulated inflammation is injurious because it results in different diseases such as rheumatoid arthritis, septic shock and cancer (1). Apart from the stimulus, inflammation is involved in an adaptive response for restoring homeostasis (1). Tissue macrophages and mast cells are involved in recognition of primary infection and lead to production of different inflammatory mediators, including pro-inflammatory cytokines and chemokines. The instantaneous effects of these mediators educe inflammatory exudates which contain plasma proteins and neutrophils (1).
In general, TLRs respond to extracellular stimuli while NLRs are involved in detection of cytosolic stimuli and provide crucial host defense against pathogens (5, 9). All TLRs recognize specific unique molecular patterns of microbes and elicit the innate immune system (10, 11). Myeloid differentiation primary response gene 88 (MyD88), toll-interleukin 1 receptor (TIR) domain containing adaptor protein (TIRAP)/MyD88 adaptor like (Mal), TIR domain-containing adapter-inducing interferon-β (TRIF), and TRIF-related adaptor molecule (TRAM) are TIR domain containing adaptors which are involved in regulation of TLR-mediated signaling pathways (12, 13). Most TLRs are involved in signaling by involving MyD88 with the exception of TLR3 which signals through TRIF. Deficiency of MyD88 leads to defects in production of IL-12 p40 and TNF-α, thus MyD88 plays an important role in regulation of innate immune response (12~14). The TRIF can also be used in combination with TRAM in the TLR4-MyD88-independent pathway (6, 15, 16). TLR3 employ TRIF to activate interferon regulated factor 3 (IRF3) which ultimately translocates to nucleus and results in expression of interferon (IFN)-β (15, 16).
Activation of TLRs results in recruitment of IL-1 receptor-associated kinases (IRAKs) family members through MyD88-dependent mechanism. The activated IRAK associates with downstream adaptor tumor necrosis factor receptor (TNFR)-associated factor 6 (TRAF6) and results in activation of TGF-β-activated kinase 1 (TAK1) (17). Activated TAK1 complex regulates IκB kinases (IKK) complex and mitogen activated protein kinases (MAPKs) which ultimately result in activation of NF-κB and AP-1, respectively (18). Finally activated NF-κB and AP-1 is responsible for production of proinflammatory cytokines including IL-12, IL-6 and TNF-α (19). The activation of NF-κB and MAPKs is tightly regulated by phosphorylation and ubiquitination and is responsible for production of TNF-α, IL-6 and IL-12 (20). Translocation of NF-κB to nucleus is also involved in expression of pro-forms of IL-1β and IL-18. Further processing and release of IL-1β and IL-18 is dependent on NLRs and caspase-1 (7, 21, 22). Recent reports have highlighted the roles of NF-κB and MAPK pathways in response to inflammatory conditions and infectious agents. Moreover, MAPKs also play critical role in regulation of inflammation-associated cancer development (23).
MAPKs were initially called as extracellular signal-regulated kinases (ERKs); a chain of proteins in the cell that is involved in communication of signals in eukaryotic cells. MAPK pathway plays critical role in communicating signals from receptors on the cell surface to the DNA in the nucleus to manage cellular functions such as proliferation, growth, differentiation, migration and death (24, 25) (Fig. 1). Binding of growth factor to the receptor on the cell surface results in initiation of signal transduction and activation of gene expression and then terminates this signal as negative feedback. Different MAPKs pathways have been characterized in mammals: ERK1 and 2, c-Jun amino-terminal kinases (JNK) 1, 2, and 3, p38 isoforms α, β, γ and δ, and ERK3 and 4, and ERK5 (26).
Various factors can activate MAPKs, but generally growth factors activate ERK1 and ERK2, whereas the JNK and p38 are activated in response to stress stimuli (27). All MAPKs have distinctive characteristics, but some features are common by the MAPK pathways and each family possesses conserved consecutively acting kinases; which includes MAPK, a MAPK kinase (MAPKK), and a MAPKK kinase (MAPKKK) (28). MAPKKK phosphorylates and activates a MAPKK, which ultimately activate MAPK through dual phosphorylation on threonine and tyrosine residues (28). MAPK not only mediates diverse range of functions by phosphorylation of phospholipases, transcription factors, and cytoskeletal proteins but also catalyzes the phosphorylation and activation of protein kinases, called MAPK-activated protein kinases (MKs) (28). The MKs are related kinases, involved in diverse range of biological functions and play their role as amplification step in MAPK catalytic cascades. The MK family consists of the mitogen- and stress-activated kinases (MSKs), the 90-kDa ribosomal S6 kinases (RSKs), the MAPK interacting kinases (MNKs), MK2, MK3, and MK5 (28). The activity of MAPKs is managed by phosphorylation of two residues; a threonine and a tyrosine, found in phosphorylation lip or activation loop (29, 30).
The mammalian ERK1 and ERK2 (p44 and p42 MAPK) have more than 80% amino acid identity and copiously present in all tissues (27). Rapidly accelerated fibrosarcoma (Raf) kinases are a family of three serine/threonine-specific protein kinases, A-Raf, B-Raf, C-Raf, and play important role in the Ras-Raf-MEK-ERK signal transduction cascade (31). Rat sarcoma (Ras) is a guanosine-nucleotide-binding protein and function as binary signaling switches with "on" and "off" states (32). Stimulation of MAPKKK (A-Raf, B-Raf, Raf-1 and C-Mos) by mitogen directs the phosphorylation and activation of a MAPKK (MEK1 and MEK2) which then stimulates ERK1 and ERK2 activity through dual phosphorylation on tyrosine and threonine residues.
Stimulation of cell surface receptors such as tyrosine kinases and G protein-coupled receptors induce signal and activate Raf/MEK/ERK cascade (33). Activation of Raf leads to phosphorylation of MEK1 and MEK2 which ultimately phosphorylate ERK1 and ERK2 (34). ERK1/2 signaling plays an important role in regulation of cell proliferation. Activation of ERK1 and ERK2 leads to phosphorylation of different substrates which include some membrane proteins, cytoskeletal proteins, nuclear substrates and numerous MKs (26). In ear edema of mouse the activities of both ERK-1 and ERK-2 were upregulated and their phosphorylation was measurable after 15 minutes. Inhibition of MAPK/ERK pathway has been successfully used in treating inflammation in an ear edema of mice (35).
JNKs are also called as stress-activated kinases (SAPKs), because initially they were characterized by their activation in response to cell stress (36, 37). Initially the first member of the JNK family was isolated from rat liver (38). JNK1, JNK2, and JNK3 are also called as SAPK γ, SAPK α, and SAPK β, respectively (27, 36). The JNKs can be activated by UV irradiation, growth factor deprivation, cytokines and DNA-damaging agents. All the JNKs are ubiquitously expressed; however, JNK3 is mainly expressed in brain, heart and testes (36, 39~42). Activation of MAPKKK (MEKK1-4, DLK, MLK2 Tpl-2, ASK1, TAK1 and TAO1/2) by stimuli results in activation of MAPKK, which includes MEK4 and MEK7 and are responsible for the activation of JNKs. The activation of JNK necessitates dual phosphorylation on tyrosine and threonine residues within a conserved Thr-Pro-Tyr (TPY) motif (36).
The transcription factor c-Jun is known as a substrate for JNKs. The JNKs are also involved in phosphorylation of other transcription factors which include activating transcription factor-2 (ATF-2), nuclear factor of activated T-cells cytoplasmic 1 (NF-ATc1), heat shock factor protein 1 (HSF-1), and Signal transducer and activator of transcription 3 (STAT3) (26, 36, 43). Roles of MK activated by JNKs still remain unclear. The activator protein 1 (AP-1) is a transcription factor which controls gene expression and can be activated by a variety of stimuli, including cytokines, stress, growth factors and infections (44). Phosphorylation of c-Jun, ATF2 and ATF3 substrates by JNKs results in enhancement of AP-1 controlled specific gene expressions (45). AP-1 is involved in managing several cellular processes such as proliferation, differentiation and apoptosis (46).
Four p38 isoforms are found in mammals, which include: p38α, p38β, p38γ, and p38δ. Most of the tissues widely express p38α and p38β isoforms, whereas p38γ, and p38δ are expressed by kidney, skin and muscle cells (47). Defect in p38 was proposed to be involved in impaired vascularization of the placenta and results in embryonic death at the age of 10.5~16.5 in mice (48, 49). The p38 is also involved in insulin secretion in pancreatic β cells, as mice lacking p38 were found to be responsible for defects in insulin secretion (50). The p38 isoforms are strongly activated by stress and inflammatory cytokines in mammalian cells (51). The p38α gives response to heat shock and endotoxic lipopolysaccharide (47). The phosphorylation of p38 is managed by MKK3 and MKK6 which are under upstream control of MTK1 and ASK1 (28, 52). Activation of p38 results in phosphorylation of various cellular targets which include the microtubule-associated protein Tau, cytosolic phospholipase A2, and the transcription factors ATF1, ATF2, MEF2A, Sap-1, Elk-1, NF-κB, Ets-1, and p53. The p38 is also involved in activation of some MKs which include MSK1 and MSK2, MNK1 and MSK2 (36).
Roles of TLRs and MAPKs in inflammation have been well characterized. All TLRs recognize distinctive PAMPs by involving some intracellular adaptors and MAPKs lead to expression of response to stimuli. MAPK signaling cascades are involved in regulation of key cellular functions which include cell proliferation, gene expression, cell motility and cell survival or death. A wide range of inhibitors of MAPK signaling pathways are available and scientists are working on identifying more natural MAPK inhibitors which will be helpful in treating inflammatory conditions and cancer.
Figures and Tables
Figure 1
MAPK signaling pathways. The MAPK signaling pathway is stimulated by stress, growth factors, pathogen-associated molecular patterns and inflammatory cytokines. Stimulation of MAPKKK (A-Raf, B-Raf, and C-Raf) leads to the phosphorylation and activation of a MAPKK (MEK1/2) which then stimulates MAPK (ERK1/2) activity. Activation of ASK1, TAK1, and MEKK1/2 by stimuli results in activation of MEK4/7 which are responsible for the activation of JNKs. The phosphorylation of p38 is managed by MKK3/6 which are under upstream control of MTK1 and ASK1. Activation of TAK1 subsequently induces activation and translocation of NF-κB to nucleus. ERK1/2, JNK, and p38 are responsible for activation of transcription factors including TCF/ELK1, ATF-2 and AP-1, and others. Various transcription factors including these lead to expression of genes encoding inflammatory cytokines, cell differentiation, growth and apoptosis. TFs, transcription factors; GF, growth factor; GFR, growth factor receptor; TLR, Toll-like receptor; PAMPs, pathogen-associated molecular patterns; TNF, tumor necrosis factor; TNFR, tumor necrosis factor receptor.
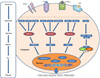
References
3. O'Neill LA, Bowie AG. The family of five: TIR domain-containing adaptors in Toll-like receptor signalling. Nat Rev Immunol. 2007. 7:353–364.
4. Trinchieri G, Sher A. Cooperation of Toll-like receptor signals in innate immune defence. Nat Rev Immunol. 2007. 7:179–190.


5. Miao EA, Leaf IA, Treuting PM, Mao DP, Dors M, Sarkar A, et al. Caspase-1-induced pyroptosis is an innate immune effector mechanism against intracellular bacteria. Nat Immunol. 2010. 11:1136–1142.


7. Hong S, Park S, Yu JW. Pyrin domain (PYD)-containing inflammasome in innate immunity. J Bacteriol Virol. 2011. 41:133–146.


8. Manzoor Z, Koh YS. Caspase-11, the main executioner in non-canonical inflammasome. J Bacteriol Virol. 2012. 42:169–171.


9. Medzhitov R. Recognition of microorganisms and activation of the immune response. Nature. 2007. 449:819–826.


10. Ishii KJ, Koyama S, Nakagawa A, Coban C, Akira S. Host innate immune receptors and beyond: making sense of microbial infections. Cell Host Microbe. 2008. 3:352–363.


11. Koh YS. Nucleic acid recognition and signaling by Toll-like receptor 9: compartment-dependent regulation. J Bacteriol Virol. 2011. 41:131–132.


12. Takeda K, Akira S. Toll-like receptors in innate immunity. Int Immunol. 2005. 17:1–14.
13. Pålsson-McDermott EM, O'Neill LA. Building an immune system from nine domains. Biochem Soc Trans. 2007. 35:1437–1444.


14. Hemmi H, Kaisho T, Takeuchi O, Sato S, Sanjo H, Hoshino K, et al. Small anti-viral compounds activate immune cells via TLR7 MyD88-dependent signaling pathway. Nat Immunol. 2002. 3:196–200.


15. Yamamoto M, Sato S, Hemmi H, Hoshino K, Kaisho T, Sanjo H, et al. Role of adaptor TRIF in the MyD88-independent toll-like receptor signaling pathway. Science. 2003. 301:640–643.


16. Yamamoto M, Takeda K, Akira S. TIR domain-containing adaptors define the specificity of TLR signaling. Mol Immunol. 2004. 40:861–868.


18. Kawai T, Akira S. Signaling to NF-kappa B by Toll-like receptors. Trends Mol Med. 2007. 13:460–469.
19. Kawai T, Akira S. The role of pattern-recognition receptors in innate immunity: update on Toll-like receptors. Nat Immunol. 2010. 11:373–384.


20. Sun SC, Ley SC. New insights into NF-kappa B regulation and function. Trends Immunol. 2008. 29:469–478.
21. Miao EA, Andersen-Nissen E, Warren SE, Aderem A. TLR5 and Ipaf: dual sensors of bacterial flagellin in the innate immune system. Semin Immunopathol. 2007. 29:275–288.


22. Kayagaki N, Warming S, Lamkanfi M, Vande Walle L, Louie S, Dong J, et al. Non-canonical inflammasome activation targets caspase-11. Nature. 2011. 479:117–121.


23. Wagner EF, Nebreda AR. Signal integration by JNK and p38 MAPK pathways in cancer development. Nat Rev Cancer. 2009. 9:537–549.


24. Huang P, Han J, Hui L. MAPK signaling in inflammation-associated cancer development. Protein Cell. 2010. 1:218–226.


25. Martinez E. Multi-protein complexes in eukaryotic gene transcription. Plant Mol Biol. 2002. 50:925–947.
26. Chen Z, Gibson TB, Robinson F, Silvestro L, Pearson G, Xu B, et al. MAP kinases. Chem Rev. 2001. 101:2449–2476.


27. Pearson G, Robinson F, Beers Gibson T, Xu BE, Karandikar M, Berman K, et al. Mitogen-activated protein (MAP) kinase pathways: regulation and physiological functions. Endocr Rev. 2001. 22:153–183.


28. Roux PP, Blenis J. ERK and p38 MAPK-activated protein kinases: a family of protein kinases with diverse biological functions. Microbiol Mol Biol Rev. 2004. 68:320–344.


29. Blank JL, Gerwins P, Elliott EM, Sather S, Johnson GL. Molecular cloning of mitogen-activated protein/ERK kinase kinases (MEKK) 2 and 3. Regulation of sequential phosphorylation pathways involving mitogen-activated protein kinase and c-Jun kinase. J Biol Chem. 1996. 271:5361–5368.


30. Chou MM, Hanafusa H. A novel ligand for SH3 domains. The Nck adaptor protein binds to a serine/threonine kinase via an SH3 domain. J Biol Chem. 1995. 270:7359–7364.
31. Zebisch A, Troppmair J. Back to the roots: the remarkable RAF oncogene story. Cell Mol Life Sci. 2006. 63:1314–1330.


32. Hall A, Marshall CJ, Spurr NK, Weiss RA. Identification of transforming gene in two human sarcoma cell lines as a new member of the ras gene family located on chromosome 1. Nature. 1983. 303:396–400.


33. Campbell SL, Khosravi-Far R, Rossman KL, Clark GJ, Der CJ. Increasing complexity of Ras signaling. Oncogene. 1998. 17:1395–1413.


34. Hallberg B, Rayter SI, Downward J. Interaction of Ras and Raf in intact mammalian cells upon extracellular stimulation. J Biol Chem. 1994. 269:3913–3916.


35. Jaffee BD, Manos EJ, Collins RJ, Czerniak PM, Favata MF, Magolda RL, et al. Inhibition of MAP kinase kinase (MEK) results in an anti-inflammatory response in vivo. Biochem Biophys Res Commun. 2000. 268:647–651.


36. Kyriakis JM, Avruch J. Mammalian mitogen-activated protein kinase signal transduction pathways activated by stress and inflammation. Physiol Rev. 2001. 81:807–869.


37. Johnson GL, Nakamura K. The c-Jun kinase/stress-activated pathway: Regulation, function and role in human disease. Biochim Biophys Acta. 2007. 1773:1341–1348.


38. Kyriakis JM, Avruch J. pp54 microtubule-associated protein 2 kinase. A novel serine/threonine protein kinase regulated by phosphorylation and stimulated by poly-L-lysine. J Biol Chem. 1990. 265:17355–17363.


39. Yang DD, Kuan CY, Whitmarsh AJ, Rincón M, Zheng TS, Davis RJ, et al. Absence of excitotoxicity-induced apoptosis in the hippocampus of mice lacking the Jnk3 gene. Nature. 1997. 389:865–870.


40. Dérijard B, Hibi M, Wu IH, Barrett T, Su B, Deng T, et al. JNK1: a protein kinase stimulated by UV light and Ha-Ras that binds and phosphorylates the c-Jun activation domain. Cell. 1994. 76:1025–1037.


41. Kyriakis JM, Banerjee P, Nikolakaki E, Dai T, Rubie EA, Ahmad MF, et al. The stress-activated protein kinase subfamily of c-Jun kinases. Nature. 1994. 369:156–160.


42. Pulverer BJ, Kyriakis JM, Avruch J, Nikolakaki E, Woodgett JR. Phosphorylation of c-jun mediated by MAP kinases. Nature. 1991. 353:670–674.


43. Akira S, Nishio Y, Inoue M, Wang XJ, Wei S, Matsusaka T, et al. Molecular cloning of APRF, a novel IFN-stimulated gene factor 3 p91-related transcription factor involved in the gp130-mediated signaling pathway. Cell. 1994. 77:63–71.


44. Hess J, Angel P, Schorpp-Kistner M. AP-1 subunits: quarrel and harmony among siblings. J Cell Sci. 2004. 117:5965–5973.


46. Ameyar M, Wisniewska M, Weitzman JB. A role for AP-1 in apoptosis: the case for and against. Biochimie. 2003. 85:747–752.


47. Hui L, Bakiri L, Stepniak E, Wagner EF. p38alpha: a suppressor of cell proliferation and tumorigenesis. Cell Cycle. 2007. 6:2429–2433.


48. Allen M, Svensson L, Roach M, Hambor J, McNeish J, Gabel CA. Deficiency of the stress kinase p38alpha results in embryonic lethality: characterization of the kinase dependence of stress responses of enzyme-deficient embryonic stem cells. J Exp Med. 2000. 191:859–870.
49. Mudgett JS, Ding J, Guh-Siesel L, Chartrain NA, Yang L, Gopal S, et al. Essential role for p38alpha mitogen-activated protein kinase in placental angiogenesis. Proc Natl Acad Sci U S A. 2000. 97:10454–10459.


50. Sumara G, Formentini I, Collins S, Sumara I, Windak R, Bodenmiller B, et al. Regulation of PKD by the MAPK p38delta in insulin secretion and glucose homeostasis. Cell. 2009. 136:235–248.

