Abstract
This study was to determine the effects of allogenic umbilical cord blood (UCB)-derived mesenchymal stem cells (MSCs) and recombinant methionyl human granulocyte colony-stimulating factor (rmhGCSF) on a canine spinal cord injury model after balloon compression at the first lumbar vertebra. Twenty-five adult mongrel dogs were assigned to five groups according to treatment after a spinal cord injury: no treatment (CN); saline treatment (CP); rmhGCSF treatment (G); UCB-MSCs treatment (UCB-MSC); co-treatment (UCBG). The UCB-MSCs isolated from cord blood of canine fetuses were prepared as 106 cells/150 µl saline. The UCB-MSCs were directly injected into the injured site of the spinal cord and rmhGCSF was administered subcutaneously 1 week after the induction of spinal cord injury. The Olby score, magnetic resonance imaging, somatosensory evoked potentials and histopathological examinations were used to evaluate the functional recovery after transplantation. The Olby scores of all groups were zero at the 0-week evaluation. At 2 week after the transplantation, the Olby scores in the groups with the UCB-MSC and UCBG were significantly higher than in the CN and CP groups. However, there were no significant differences between the UCB-MSC and UCBG groups, and between the CN and CP groups. These comparisons remained stable at 4 and 8 week after transplantation. There was significant improvement in the nerve conduction velocity based on the somatosensory evoked potentials. In addition, a distinct structural consistency of the nerve cell bodies was noted in the lesion of the spinal cord of the UCB-MSC and UCBG groups. These results suggest that transplantation of the UCB-MSCs resulted in recovery of nerve function in dogs with a spinal cord injury and may be considered as a therapeutic modality for spinal cord injury.
Regeneration of the central nervous system is limited after injuries related to ischemia, trauma and degenerative disease [33]. Recently, cell transplantation therapy has been considered for treatment in the field of regenerative medicine [3,27]. At the early stages of these studies, neural stem cells were obtained mainly from fetal tissue to explore their use for regeneration [5]. However, due to their limited availability alternative sources of cells for neural transplantation, such as embryonic, bone marrow, adipose and umbilical cord blood (UCB) stem cells are being evaluated [27,[30,[41]. The cells obtained from these additional sources can survive, proliferate, migrate, and differentiate into neuronal phenotypes in the damaged brain and spinal cord [1,[21,[34,40].
UCB stem cells, used for cell therapy, have advantages over the use of other sources of stem cells [4]. UCB cells have more mesenchymal progenitor cells per volume, and are more pluripotent and genetically flexible than bone marrow-derived mesenchymal stem cell [4,7]. In addition, it has been suggested that they are not as mature as other adult stem cells so that they may not elicit alloreactive responses that modulate the immune system [2,13,16,37]. Furthermore, they have been shown to have lower carcinogenic potential than embryonic stem cells [15].
Recently, UCB stem cells have been shown to differentiate into neural cells in vitro [7,8]. In addition, functional recovery after transplantation of UCB stem cells into an injured area of the spinal cord in vivo [40] and the efficacy of intravenous administration of UCB stem cells in traumatic brain injury have been demonstrated [20]. Furthermore, there has been a report on recovery after transplantation of UCB stem cells with neurotrophic factors [15]. The treatment with granulocyte colony-stimulating factor (GCSF) has been shown to stimulate bone marrow stem cell proliferation and mobilization [25].
Although canine bone marrow-derived mesenchymal stem cells have been isolated and characterized in many studies, there are only a few reports on the isolation of mesenchymal stem cells from canine cord blood (UCB-MSCs) [22,34]. In this study, using a previously developed modified canine acute spinal cord injury model [19], we examined the effectiveness of canine UCB-MSCs and recombinant methionyl human GCSF (rmhGCSF) on the improvement of neurological function.
Twenty-five healthy adult mongrel dogs that weighed 3.77 ± 0.59 kg, were used for the experimental spinal cord injury study. This investigation was performed according to the guidelines of the 'Guide for the Care and Use of Laboratory Animals' of Seoul National University. The dogs were assigned, without bias, to five groups according to treatment after spinal cord injury. The control group with no treatment (CN: n = 5); the control group with media-injection (CP: n = 5); the group with subcutaneous injection of rmhGCSF (G: n = 5); the group with transplantation of canine UCB-MSCs into the spinal cord injury site (UCB-MSC: n = 5); the groups with transplantation of canine UCB-MSCs and subcutaneous injection of rmhGCSF (UCBG: n = 5).
The spinal cord injury (SCI) was performed using balloon compression methods as described previously [19]. Briefly, the dogs were anesthetized with intravenous administration of diazepam (Melode; Dong Wha Pharm, Korea) at a dose of 0.3 mg/kg and propofol (Anepol; Ha Na Pharm, Korea) at 6 mg/kg with atropine sulfate (Atropine; Je Il Pharm, Korea) at 0.05 mg/kg subcutaneously. Anesthesia was maintained by inhalation of 2% isoflurane (Aerane; Ilisung, Korea). Datex-Ohmeda (Microvtec Display, UK) was used for monitoring of physiologic measures including rectal temperature, oxygen saturation and pulse rate during anesthesia. The dogs were placed in a ventral recumbent position. The hemilaminectomy was performed by a left paramedian approach at L4. A three to five millimeter hole was made in the left vertebral arch at L4 using a high-speed pneumatic burr. A three-French embolectomy catheter (SORIN Biomedica, Italy) was inserted into the hole made at the L4 vertebral arch. The balloon was advanced, under fluoroscopic guidance, until the tip of the catheter was placed at the cranial margin of the L1 vertebral body. The balloon was then inflated 150 µl by injection of a contrast agent (Omnipaque; Amersham Health, Ireland) diluted 50 : 50 with saline. The soft tissues and skin were closed as per standard methods. The balloon was fixed with a Chinese finger type suture, and then removed after 12 h. After the operation, the dogs were monitored in an intensive care unit, and if needed, manual bladder expression was performed at least three times daily until voluntary urination was established.
UCB was obtained during the Caesarian section of a 58 kg mongrel dog at Seoul National University Veterinary Medical Teaching Hospital. Eight ml of blood was collected using 10 ml plastic syringes that contained 2 ml of citrate-phosphate-dextrose anticoagulant.
The low-density mononuclear cells were isolated using Ficoll-Plaque Plus (Amersham Biosciences, Sweden). Then, the cells were cultured in growth medium [Dulbeco's Modified Eagle media-low glucose with the addition of 10% fetal bovine serum (Gibco-BRL, USA) with 2mmol/l L-glutamine (Gibco-BRL, USA)] and 0.3% penicillin-streptomycin (Gibco-BRL, USA) at 37℃ and 5% CO2 concentration [26]. The UCB-MSCs were cultured and the mononucleated cells that proliferated from the cord blood were characterized by FACS analysis [11]. The cells were prepared as 1 × 106 in 150 µl of saline solution for the injection.
Transplantation of canine UCB-MSCs was performed a week after the SCI. The dogs were anesthetized using the same methods described above. The CN group did not have any cells or medium transplanted after the injury. In the CP group, a laminectomy was performed and the injured site was exposed by a durotomy. One hundred and fifty micro liters of saline was injected into the spinal cord at three locations (center of the injury, 1.0 mm proximal and 1.0 mm distal to the injury, 3.0 mm in depth) using a 30 gauge needle. The incision region of the dura mater was sutured with 8.0 absorbable materials. The remaining lesion was closed routinely. In the UCB-MSCs group, 1 × 106 of cells suspended in 150 µl of saline solution were injected at the SCI site. In the UCBG group, 1 × 106 of cells suspended in 150 µl of saline solution were injected at the SCI site and 100 µl of rmhGCSF solution (GCSF; Dong Wha Pharm, Korea) was injected subcutaneously for 7 days, once a day. The rmhGCSF was prepared with 5 µg/animal/day and diluted with sterilized saline. In group G, 100 µl of rmhGCSF solution was injected using the same method as in the UCBG group.
Behavioral assessment was performed to evaluate the functional recovery of the hind limbs. Each dog was videotaped for a minimum of 10 steps from both sides and behind when walking on the floor. Dogs with non-weight bearing on their hind limbs were also videotaped, supported by holding the base of their tail. Using a 15-point scoring system (Olby score), the dogs' gait was independently scored from the videotapes by two individuals unaware of the experimental conditions [24]. A mean score at 0, 2, 4, and 8 weeks after the SCI was calculated.
Somatosensory evoked potentials (SEP) were measured using the Neuropack 2 (Nihon Kohden, Japan) and two subdermal channels at 0, 4 and 8 weeks after the cell transplantation. Channel 1 was located in the subdermal region at the midline between the sixth and seventh lumbar vertebra and channel 2 was positioned between the tenth and eleventh thoracic vertebra using platinum needle Grass stimulating electrodes (Astro-Med, USA). The posterior tibial nerve was stimulated for 0.2 msec, with 2 Hz and 3 mA [39]. The latency response was converted into the velocity as a measure of spinal cord dysfunction with the evoked potentials. Spinal conduction velocity (CV) from L6~L7 to T10~T11 was calculated by the following equation [39]: Conduction velocity (m/sec) = distance (cm) of two points/latency (msec) difference × 10.
Magnetic resonance imaging (MRI) was performed with a 0.2 Tesla Magnet scanner (Esaote, Italy) at a week after the SCI and at 4 and 8 weeks after the cell injection. The majority of the images obtained were interleaved 5.0 mm with a slice thickness of 5.0 mm. The repetition time (TR) and time to echo (TE) were adjusted. T1-weighted (T1W) (TR/TE = 540/26 msec) and T2-weighted (T2W) echo (TR/TE = 380/90 msec) images were obtained. All dogs in each of the groups were examined and the spinal cord injury lesions expressed in T2W sagittal planes at 0, 4 and 8 weeks after the injury.
To assess the histopathological changes, all dogs were euthanized at 8 weeks after the cell treatment. The spinal cords from T10 to L4 of all dogs were sampled and fixed in 10% buffered neutral formalin. And then the tissues were rountinely processed, embedded and sectioned at 4 µm. These sections were mounted on silane-coated slide glass. The slides were stained with hematoxylin and eosin (H&E) to detect vacuolar formation and Luxol fast blue to identify myelin [6]. The volumes of the cavities in the damaged spinal cord were calculated from images of the transverse sections using image analyzer software (ImageJ version 1.37; National Institutes of Health, USA). The myelinated area was analyzed using the same software. These two analyses were performed at the epicenter of the damaged spinal cord.
The Olby scores for the degree of nerve function, in all groups, was zero at 0 week. The Olby scores in the groups UCB-MSC, G and UCBG were increased 1.2, 0.8 and 1.4 at 2 weeks after treatment and 7.4, 3.0 and 4.2 at 8 weeks, respectively (Fig. 1). However, the increase in the Olby scores in the CN and CP groups was below 1.0 until 8 weeks. The Olby scores in the UCB-MSC and UCBG groups were significantly higher than in the CN and CP groups from 2 weeks onward after treatment. However, there were no significant differences between the UCB-MSC and UCBG groups and between the CN and CP groups. Group G did not show any significant difference from the other groups at 2 weeks. However, group G had significantly lower Olby scores than the UCB-MSC and UCBG groups at 4 and 8 weeks, although there were no differences between the UCB-MSC and UCBG groups at this time.
The somatosensory evoked potentials of the posterior tibial nerves at the thoracic level revealed no response in all groups at 0 week (Table 1). At 4 weeks, it was possible to measure the evoked potentials in the G, UCB-MSC and UCBG groups. However, the CP and CN showed no responses until 8 weeks. The conduction velocities in G, UCB-MSC and UCBG at 8 weeks were improved up to 43.92 ± 37.77 m/s, 48.92 ± 26.13 m/s and 39.64 ± 30.99 m/s, respectively.
Most dogs in all of the groups showed clear hyperintense signals in the T2W sagittal plane at the L1 lesion at 1 week after the SCI (Fig. 2). The CN and CP groups had a similar appearance at the injured site that became a clearer hyperintense area, in the parenchyma at the L1 level, until 9 weeks after the SCI. The hyperintense area in the T2W sagittal plane at the L1 level was decreased in two dogs in the group G at 8 weeks. One of the dogs in the UCBG group had a slightly decreased hyperintense lesion in the T2W sagittal plane at the L1 level. In the UCB-MSC group, one dog showed a normointense intramedullar lesion but it was narrower than the near normal appearing areas.
At 8 weeks after treatment, the margins of normal grey and white matter were not identified in all of the dogs studied. There was a generalized infiltration of fibrous tissue and adhesions in the dura mater. Most of the dogs in the CN and CP groups showed damaged tissues and severe vacuolar formation. Cavity formation and Luxol fast blue positive area were very small in the CP group (Fig. 3A & B ). The G group showed severe crushing damage in both the white and grey matters as well as cavity formation (Fig. 3C & D ). Although the UCB-MSC group showed similar histological findings with the other groups, neuronal cell like structures in a small area were observed (Fig. 3E-G). The mean percentage of cavities in the CN, CP, G, UCB-MSC and UCBG groups were 41.06 ± 10.39, 2.64 ± 4.55, 21.37 ± 17.13, 6.55 ± 4.69, and 16.30 ± 11.46, respectively (p < 0.05) (Table 2). The CN group showed a significantly higher percentage of cavity formation. The mean percentages of areas stained with Luxol fast blue in the CN, CP, G, UCB-MSC, and UCBG groups were 13.70 ± 9.27, 8.39 ± 9.63, 30.39 ± 13.65, 46.33 ± 7.02, and 33.87 ± 25.33, respectively (p < 0.05).
The Olby scoring system was used for quantitative evaluation of functional outcomes in this study. Olby et al. modified the BBB open field scoring system for dogs based on the pelvic limbs [24]. Several investigators have confirmed the reliability of the Olby scoring system [23,38]. The spinal cord injury model in the present study had more than 75% of the spinal canal occluded during a 12 h period; the resulting lesions showed histopathologically severe hemorrhage and vacuolar formation. The dogs had paraplegia and were not expected to regain a normal gait without treatment [6] as demonstrated in the control groups, CN and CP, with or without saline injection. In the CN and CP groups, the dogs had Olby scores below one until 9 weeks after the injury. However, in the group with UCB-MSCs the Olby scores increased from 2 weeks and were over 6 at 8 weeks; the weight-bearing of the pelvic limbs improved from 10 to 50% of the time. Therefore, the group with UCB-MSCs appeared to have improved spinal cord function after the experimentally induced spinal cord injury.
A severe spinal cord injury results in the formation of glial scar rimming, a cavitation that becomes a fluid-filled cyst [33]. This fluid filled cyst may be bypassed by building new connections with new neurons provided by the differentiation of exogenous cells [35]. If guidance is provided, with a bridge or scaffold made up of various cells or materials, for connection to the distal and proximal ends of the spinal cord, the injured cord may offer support for the transplanted cells to facilitate regeneration [35,40]. Although we did not confirm the fate of the transplanted stem cells, we found a smaller percentage of cavity formation in the UCB-MSC and UCBG groups. In addition, the CP group demonstrated the smallest percentage of cavity formation; however, a few myelinated areas remained compared with the UCB-MSC and UCBG groups. Our histopathology data suggested that transplantation of the UCB-MSCs might improve the functional outcome of spinal cord injury by creating new neuronal pathways in the fibrous scar tissues.
In the present study, the UCB-MSC group showed significantly higher Olby scores than the G group from 4 weeks after cell transplantation. There was no difference between the UCB-MSC and UCBG groups and between the UCBG and G groups. Our results suggest that GCSF had no beneficial effect on the spinal cord injury. However, it was reported that GCSF could stimulate bone marrow stem cells to proliferate and mobilize [25]. The GCSF stimulates neurogenesis via the vascular endothelial growth factor [12]. The GCSF enhances angiogenesis by increases of endothelial proliferation, vascular surface area and vascular branch points after focal CNS ischemia [18]. The angiogenic effects of GCSF reduce the function of the blood brain barrier after central nervous system disruption [14,25]. It has been shown that GCSF, under certain circumstances, such as when combined with multiple doses of cytotoxic agents, may have adverse effects on stem cells including direct damage to stem cells [36]. The UCBG group was not exposed to any cytotoxic agents in this study. We administered the GCSF by a different route for 7 days, which might have affected the UCB-MSCs. There is the possibility of a down regulation of the proliferation of the UCB-MSCs related with the administration of GCSF.
Percutaneous recording of electrical activity, in the spinal cord, is an accurate method for spinal cord function assessment [28]. The conduction velocities calculated from SEP amplitudes and latencies revealed the cord damage severity in the experimentally induced SCI [29]. Generally, the SEP has a flat waveform when the spinal cord is injured by more than fifty percent [17]. In this study, the neurological status of the CN and CP groups was consistent with a flat waveform until 8 weeks. However, the values of the SEP recordings were similar even though a different clinical status was shown in the G, UCB-MSC and UCBG groups. Their measurement revealed that a minimal connection was responsible for the values in the recording. However, these values did not discriminate the various clinical grades observed.
When the MR images were compared to the pathology specimens, the spindle-shape of the high intensity area in the spinal cord on the T2W images corresponded to the fibrous area, and the low intensity areas reflected cavity formation in the chronic phase [6]. In the present study, there were markedly hyper- or hypo-intense areas in the CN and CP groups after 8 weeks. However, all of the lesions in the UCB-MSC and UCBG groups had a decrease of the hyper-intense areas after 8 weeks. Similar findings have been reported in severe human spinal cord injuries [13].
Neural stem cells isolated from embryonic and adult brain tissues, and both mouse and human umbilical cord blood derived multipotent stem cells, have been used for repair of central nervous system injury [27,[40,[41]. Adipose-derived stem cells have been reported to have a therapeutic potential for neurological disorders [31]. It is unclear what the mechanism is by which allogenic UCB-MSCs transplantation induces recovery of spinal cord function. In a rat model, it was reported that the stem cells expressed glial fibriliary acidic protein or neuronal nuclear antigens [40]. The stem cells have the characteristic of specific homing and the expression of inflammatory molecules including adhesive molecules, chemokines, cytokines, and chemokine receptors at the injured site [4]. Tracking studies of MSCs, in vivo, with MRI or immunohistochemistry have demonstrated the fate of cells; these cells have been observed to integrate into the areas with lesions in the central nervous system [10,32]. In addition, endogeneous neural progenitor cells may be activated by injury and rapidly divide to replace damaged cells [9].
Although the results of this study showed that there was evidence of functional and sensory improvement after allogenic UCB-MSCs transplantation, there was no evidence of regeneration of spinal cord tissue by magnetic resonance imaging and histology. However, there was no additional damage to the experimentally injured spinal cord such as inflammatory responses after direct transplantation into the cord with allogenic UCB-MSCs. In addition, we observed new neuronal formation in the injured structures of the spinal cord in the UCB-MSC and UCBG groups. Therefore, the results of this study showed that transplantation of UCB-MSCs resulted in recovered nerve function in dogs after a spinal cord injury. Such transplantation procedures may provide a good therapeutic option for recovery after spinal cord injuries. Further investigation on the fate of UCB-MSCs after transplantation is needed to confirm the role of stem cells in the regeneration of the spinal cord after an injury.
Figures and Tables
Fig. 1
Olby scores during 8 weeks. The groups of UCB-MSC and UCBG improved more as shown with their functional scores compared with the groups of CN and CP at 2 week after transplantation (p < 0.05). The UCBG and UCB-MSC groups were significantly improved as compared with all other groups at 8 week after transplantation (p < 0.05).
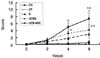
Fig. 2
Magnetic resonance images of the spinal cord in T2W sagittal view of the group CN (A & B), CP (C & D), G (E & F), UCB-MSC (G & H) and UCBG (I & J). Most of the dogs were shown the clear localization of the spinal cord injury lesion (circle). A, C, E, G and I: Before cell transplantation, 1 week after spinal cord injury. B, D, F, H and J: 8 weeks after cell transplantation.
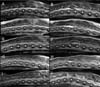
Fig. 3
Histopathological findings at 8 weeks after cell transplantation. (A) The epicenter of injured spinal cord of a dog in the group CP, which showed small cavity formation with a little Luxol fast blue positive area. Luxol fast blue stain. Counterstain with cresyl violet. ×12.5. (B) High magnification of A. ×40. (C) The epicenter of injured spinal cord of a dog in the group G, which was crushed and damaged in both white and grey matters with cavity formation. H&E stain. ×12.5. (D) Same lesion as C. It showed small amount of remained myelin (arrow). Luxol fast blue stain. Counterstain with cresyl violet. ×12.5. (E) The epicenter of injured spinal cord of a dog in the group UCB-MSC, which revealed abnormal structures, however it showed structural consistency with nerve cell body (circle). H&E stain. ×12.5. (F) High magnification of E (circle). H&E stain. ×400. (G) Nerve cell body in the same lesion of F (arrows). Luxol fast blue stain. Counterstain with cresyl violet. ×400.
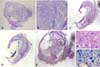
Acknowledgments
This work was supported by BK21 Program for Veterinary Science and Seoul R&BD Program (10548).
References
1. Akiyama Y, Radtke C, Kocsis JD. Remyelination of the rat spinal cord by transplantation of identified bone marrow stromal cells. J Neurosci. 2002. 22:6623–6630.


2. Di Nicola M, Carlo-Stella C, Magni M, Milanesi M, Longoni PD, Matteucci P, Grisanti S, Gianni AM. Human bone marrow stromal cells suppress T-lymphocyte proliferation induced by cellular or nonspecific mitogenic stimuli. Blood. 2002. 99:3838–3843.


3. Enomoto M, Wakabayashi Y, Qi ML, Shinomiya K. Present situation and future aspects of spinal cord regeneration. J Orthop Sci. 2004. 9:108–112.


4. Erices A, Conget P, Minguell JJ. Mesenchymal progenitor cells in human umbilical cord blood. Br J Haematol. 2000. 109:235–242.


5. Fine A. Transplantation of fetal cells and tissue: an overview. CMAJ. 1994. 151:1261–1268.
6. Fukuda S, Nakamura T, Kishigami Y, Endo K, Azuma T, Fujikawa T, Tsutsumi S, Shimizu Y. New canine spinal cord injury model free from laminectomy. Brain Res Brain Res Protoc. 2005. 14:171–180.


7. Gang EJ, Hong SH, Jeong JA, Hwang SH, Kim SW, Yang IH, Ahn C, Han H, Kim H. In vitro mesengenic potential of human umbilical cord blood-derived mesenchymal stem cells. Biochem Biophys Res Commun. 2004. 321:102–108.


8. Goodwin HS, Bicknese AR, Chien SN, Bogucki BD, Oliver DA, Quinn CO, Wall DA. Multilineage differentiation activity by cells isolated from umbilical cord blood: expression of bone, fat, and neural markers. Biol Blood Marrow Transplant. 2001. 7:581–588.


9. Horky LL, Galimi F, Gage FH, Horner PJ. Fate of endogenous stem/progenitor cells following spinal cord injury. J Comp Neurol. 2006. 498:525–538.


10. Jendelová P, Herynek V, Urdzíková L, Glogarová K, Kroupová J, Andersson B, Bryja V, Burian M, Hájek M, Syková E. Magnetic resonance tracking of transplanted bone marrow and embryonic stem cells labeled by iron oxide nanoparticles in rat brain and spinal cord. J Neurosci Res. 2004. 76:232–243.


11. Jeong YH, Lim JH, Jung CS, Kweon OK, Lee YS, Kang KS. Osteogenic potential of canine cord blood derived mesenchymal stem cell. 4th ISSCR Annual Meeting. 2006. Toronto: ISSCR;217.
12. Jung KH, Chu K, Lee ST, Kim SJ, Sinn DI, Kim SU, Kim M, Roh JK. Granulocyte colony-stimulating factor stimulates neurogenesis via vascular endothelial growth factor with STAT activation. Brain Res. 2006. 1073-1074:190–201.


13. Kang KS, Kim SW, Oh YH, Yu JW, Kim KY, Park HK, Song CH, Han H. A 37-year-old spinal cord-injured female patient, transplanted of multipotent stem cells from human UC blood, with improved sensory perception and mobility, both functionally and morphologically: a case study. Cytotherapy. 2005. 7:368–373.


14. Komine-Kobayashi M, Zhang N, Liu M, Tanaka R, Hara H, Osaka A, Mochizuki H, Mizuno Y, Urabe T. Neuroprotective effect of recombinant human granulocyte colony-stimulating factor in transient focal ischemia of mice. J Cereb Blood Flow Metab. 2006. 26:402–413.


15. Kuh SU, Cho YE, Yoon DH, Kim KN, Ha Y. Functional recovery after human umbilical cord blood cells transplantation with brain-derived neutrophic factor into the spinal cord injured rat. Acta Neurochir (Wien). 2005. 147:985–992.


16. Le Blanc K, Tammik C, Rosendahl K, Zetterberg E, Ringdén O. HLA expression and immunologic properties of differentiated and undifferentiated mesenchymal stem cells. Exp Hematol. 2003. 31:890–896.


17. Lee J, Nam T. Evaluation of spinal cord dysfunction by the somatosensory evoked potentials in dogs [Ph.D dissertation]. 2000. Seoul: Seoul National University;66–69.
18. Lee ST, Chu K, Jung KH, Ko SY, Kim EH, Sinn DI, Lee YS, Lo EH, Kim M, Roh JK. Granulocyte colony-stimulating factor enhances angiogenesis after focal cerebral ischemia. Brain Res. 2005. 1058:120–128.


19. Lim JH, Jung CS, Byeon YE, Kim WH, Yoon JH, Kang KS, Kweon OK. Establishment of a canine spinal cord injury model induced by epidural balloon compression. J Vet Sci. 2007. 8:89–94.


20. Lu D, Sanberg PR, Mahmood A, Li Y, Wang L, Sanchez-Ramos J, Chopp M. Intravenous administration of human umbilical cord blood reduces neurological deficit in the rat after traumatic brain injury. Cell Transplant. 2002. 11:275–281.


21. Mautes AEM, Liu J, Brandewiede J, Manville J, Snyder E, Schachner M. Regional energy metabolism following short-term neural stem cell transplantation into the injured spinal cord. J Mol Neurosci. 2004. 24:227–236.


22. Nakage APM, Santana AE, de Cápua MLB, Godoy AV. Characterization and quantification of blood cells from the umbilical cord of dogs. Vet Clin Pathol. 2005. 34:394–396.


23. Olby N, Harris T, Burr J, Muñana K, Sharp N, Keene B. Recovery of pelvic limb function in dogs following acute intervertebral disc herniations. J Neurotrauma. 2004. 21:49–59.


24. Olby NJ, De Risio L, Muñana KR, Wosar MA, Skeen TM, Sharp NJ, Keene BW. Development of a functional scoring system in dogs with acute spinal cord injuries. Am J Vet Res. 2001. 62:1624–1628.


25. Park HC, Shim YS, Ha Y, Yoon SH, Park SR, Choi BH, Park HS. Treatment of complete spinal cord injury patients by autologous bone marrow cell transplantation and administration of granulocyte-macrophage colony stimulating factor. Tissue Eng. 2005. 11:913–922.


26. Park KS, Lee YS, Kang KS. In vitro neuronal and osteogenic differentiation of mesenchymal stem cells from human umbilical cord blood. J Vet Sci. 2006. 7:343–348.


27. Pluchino S, Zanotti L, Deleidi M, Martino G. Neural stem cells and their use as therapeutic tool in neurological disorders. Brain Res Brain Res Rev. 2005. 48:211–219.


28. Poncelet L, Michaux C, Balligand M. Somatosensory potentials in dogs with naturally acquired thoracolumbar spinal cord disease. Am J Vet Res. 1993. 54:1935–1941.
29. Poncelet L, Michaux C, Balligand M. Study of spinal cord evoked injury potential by use of computer modeling and in dogs with naturally acquired thoracolumbar spinal cord compression. Am J Vet Res. 1998. 59:300–306.
30. Rogers I, Casper RF. Umbilical cord blood stem cells. Best Pract Res Clin Obstet Gynaecol. 2004. 18:893–908.


31. Safford KM, Rice HE. Stem cell therapy for neurologic disorders: therapeutic potential of adipose-derived stem cells. Curr Drug Targets. 2005. 6:57–62.


32. Satake K, Lou J, Lenke LG. Migration of mesenchymal stem cells through cerebrospinal fluid into injured spinal cord tissue. Spine. 2004. 29:1971–1979.


33. Schultz SS. Adult stem cell application in spinal cord injury. Curr Drug Targets. 2005. 6:63–73.


34. Suter SE, Gouthro TA, McSweeney PA, Nash RA, Haskins ME, Felsburg PJ, Henthorn PS. Isolation and characterization of pediatric canine bone marrow CD34+ cells. Vet Immunol Immunopathol. 2004. 101:31–47.


35. Teng YD, Lavik EB, Qu X, Park KI, Ourednik J, Zurakowski D, Langer R, Snyder EY. Functional recovery following traumatic spinal cord injury mediated by a unique polymer scaffold seeded with neural stem cells. Proc Natl Acad Sci USA. 2002. 99:9606.


36. van Os R, Robinson S, Sheridan T, Mauch PM. Granulocyte-colony stimulating factor impedes recovery from damage caused by cytotoxic agents through increased differentiation at the expense of self-renewal. Stem Cells. 2000. 18:120–127.


37. Watt SM, Contreras M. Stem cell medicine: umbilical cord blood and its stem cell potential. Semin Fetal Neonatal Med. 2005. 10:209–220.


38. Webb AA, Jeffery ND, Olby NJ, Muir GD. Behavioural analysis of the efficacy of treatments for injuries to the spinal cord in animals. Vet Rec. 2004. 155:225–230.


39. Yang JW, Jeong SM, Seo KM, Nam TC. Effects of corticosteroid and electroacupuncture on experimental spinal cord injury in dogs. J Vet Sci. 2003. 4:97–101.

