Abstract
Background
Methods
Figures and Tables
Fig. 1
An early stage tumor (gliomas II). The astrocytoma is located in the left insular lobe and compresses the internal capsule (Patient 5). However, there is no HSIC (left upper figure). The pyramidal tract is traced from the posterior limb of the internal capsule. The inserted table shows that ADC is higher than that of normal subject, but FA is similar. ADC, apparent diffusion coefficient; FA, fractional anisotropy; FLAIR, fluid attenuated inversion recovery; HSIC, high signal change at the level of the internal capsule in FLAIR magnetic resonance imaging.
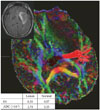
Fig. 2
A covert stage tumor [gliomas III/IV (-)]. The glioblastoma is located in the left occipital lobe with surrounding edema (Patient 14), but there was no HSIC (left upper figure). The pyramidal tract is traced from the posterior limb of the internal capsule. The inserted table shows that FA is lower and ADC is higher than in normal subjects. ADC, apparent diffusion coefficient; FA, fractional anisotropy; FLAIR, fluid attenuated inversion recovery; HSIC, high signal change at the level of the internal capsule in FLAIR magnetic resonance imaging.
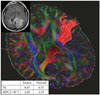
Fig. 3
An overt stage tumor [gliomas III/IV (+)]. The glioblastoma is located in the left parietal lobe with surrounding edema (Patient 23). There was HSIC (left upper figure). The pyramidal tract is traced from the posterior limb of the internal capsule. The inserted table shows that FA is lower and ADC is higher than in normal subjects. ADC, apparent diffusion coefficient; FA, fractional anisotropy; FLAIR, fluid attenuated inversion recovery; HSIC, high signal change at the level of the internal capsule in FLAIR magnetic resonance imaging.
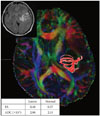
Fig. 4
FA values according to stages. FA is decreased from the covert stage (p<0.001). There is no difference associated with the presence of HSIC (glioma III/IV (-) vs. glioma III/IV (+), p=0.943). p-values are marked in the graph after the Mann-Whitney U test. FA, fractional anisotropy; FLAIR, fluid attenuated inversion recovery; HSIC, high signal change at the level of the internal capsule in FLAIR magnetic resonance imaging.
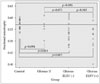
Fig. 5
ADC values according to stages. ADC increased from the early stage (p<0.001). ADC is increased if there is HSIC (p=0.031) in glioma III/IV. p-values are marked in the graph after the Mann–Whitney U test. ADC, apparent diffusion coefficient; FLAIR, fluid attenuated inversion recovery; HSIC, high signal change at the level of the internal capsule in FLAIR magnetic resonance imaging.
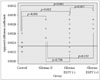
Table 1
Characteristics of the subjects
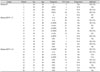
*WHO grade II gliomas, †WHO grade III or IV gliomas without HSIC, ‡WHO grade III or IV gliomas with HSIC. A-, anaplastic; EPN, ependymoma; GBL, glioblastoma; OA, oligoastrocytoma; ODG, oligodendroglioma; FLAIR, fluid attenuated inversion recovery; HSIC, high signal change at internal capsule in FLAIR MR image; N/A, not available; RT, radiotherapy; CTx, chemotherapy; F/U, follow up
Table 2
FA and ADC values in normal subjects

Left | Right | p-value* | |
---|---|---|---|
FA | 0.57±0.02 | 0.57±0.02 | 0.396 |
ADC (×10−3)† | 2.33±0.10 | 2.31±0.08 | 0.510 |
Table 3
FA and ADC values according to stages
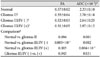
*The unit is mm2/second, †Gliomas are grouped as WHO grade II [gliomas II, early stage], grade III or IV without HSIC [gliomas III/IV (-), covert stage] and grade III or IV with HSIC [gliomas III/IV (+), overt stage], This classification reflects the stage of tumor-related white matter change, in order of increasing severity, ‡Mann-Whitney U test is used for comparison between groups. ADC, apparent diffusion coefficient; FA, fractional anisotropy; FLAIR, fluid attenuated inversion recovery; HSIC, high signal change at internal capsule in FLAIR MR image.
Acknowledgments
References





























